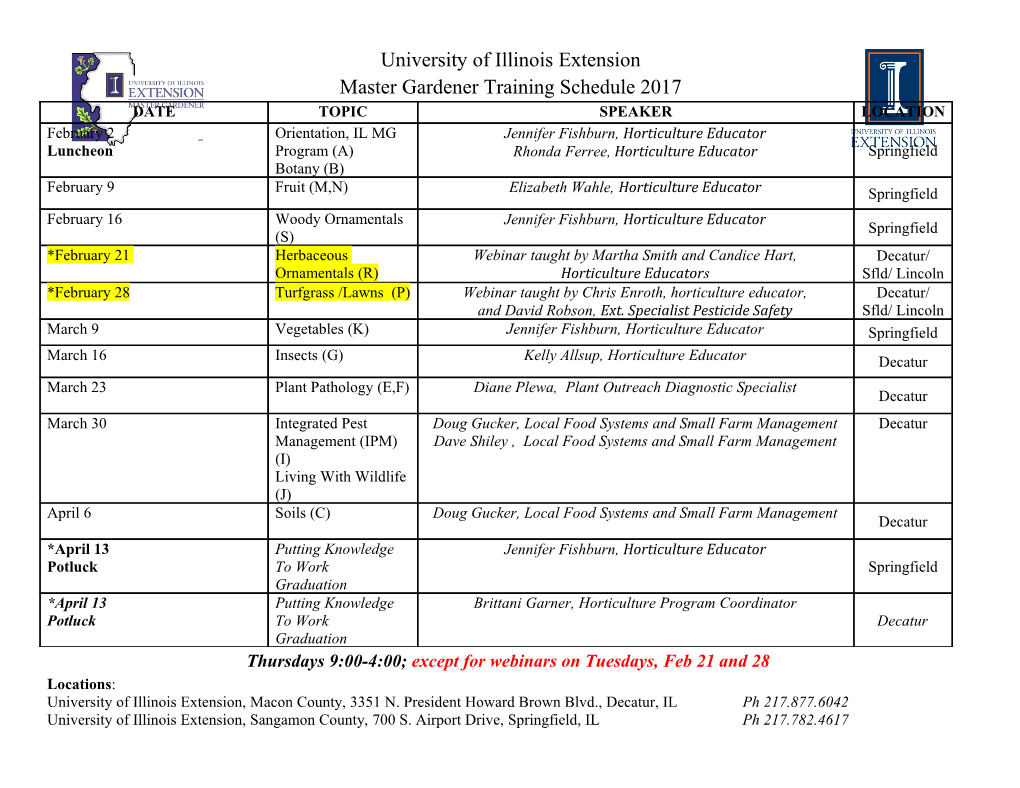
DETERMINATION OF SINGLE BASE MUTATIONS RELATED TO THE GENE SPECIFIC DISEASES BY USING ELECTROCHEMICAL DNA BIOSENSORS IN THE INTEGRATED SYSTEM Den Naturwissenschaftlichen Fakultäten der Friedrich-Alexander-Universität Erlangen-Nürnberg zur Erlangung des Doktorgrades vorgelegt von Burcu Ülker aus Izmir Als Dissertation genehmigt von den Naturwissenschaftlichen Fakultäten der Universität Erlangen-Nürnberg Tag der mündlichen Prüfung: 06.07.2005 Vorsitzender der Promotionskommission: Prof. Dr. D.-P. Häder Erstberichterstatter: Prof. Dr. Ulrich Nickel Zweitberichterstatter: Prof. Dr. Carola Kryschi Abbreviations A Adenine a Activity BSA Albumin fraction V C Cytosine CE Counter electrode σ Charge density D Diffusion coefficient DNA Deoxyribonucleic acid DPV Differential pulse voltammogram Eappl Applied potential 0 Ered ox Standard redox potential EDTA Ethylene diamine tetra acetic acid F Faraday constant (96.487 coulombs) FcII Factor II FcV Factor V G Guanine HET Heterozygote I Inosine IHP Inner Helmholz Plane iRs Ohmic potential j Current density (current per unit area, A/cm2) J Flux 2 j0 Exchange current density (A/cm ) µ i Chemical potential µ* i Electrochemical potential MUT Mutated n Number of electrons NaAc Sodium acetate buffer NAP 1-Naphthylphosphate NC Non-complementary NOS N-oxysuccinimide esters OHP Outer Helmholz Plane PCR Polymerase chain reaction pNPP p-Nitrophenylphosphate QMT Nexterion hybridization buffer R Universal gas constant (8.314 JK-1mol-1) RE Reference electrode RNA Ribonucleic acid RT Room temperature SDS Sodium dodecyl sulphate Silane 3-Glycidyloxypropyl-trimethoxysilane SPC Screen printed chip SPE Screen printed electrode SSC Sodium saline citrate T Thymine T Temperature TBS Tris buffered saline WB Washing buffer WE Working electrode WT Wild type η Overpotential φ Galvanic potential 1 Introduction 9 2 Theoretical Information 11 2.1 Electroanalytical Chemistry 11 2.1.1 Preface 11 2.1.2 Faradaic and Non-faradaic Processes 11 2.1.3 Electrochemical Cell 12 2.1.4 Electrode Reactions 12 2.1.5 Nernst Equation 14 2.1.6 Electrical Double Layer 15 2.1.7 The Electrode Set-up 16 2.1.8 Mass Transport 18 2.1.9 Overpotential 20 2.1.10 Controlled Potential Techniques 21 2.1.11 Working Electrodes 23 2.2 Nucleic Acids 24 2.2.1 Structure of Nucleic Acids 24 2.2.2 Hybridization and Denaturation 26 2.2.3 Mutations 27 2.3 Nucleic Acid Diagnostics 27 2.3.1 Preface 27 2.3.2 Polymerase Chain Reaction 28 2.3.3 Biosensors 30 2.3.4 Electrochemical DNA Biosensors 30 3 Experimental 31 3.1 Chemicals and Solutions 31 3.1.1 Chemicals 31 3.1.2 Solutions 32 3.2 Measurement Set-ups 35 3.2.1 Electrochemical Measurement Set-ups 35 3.2.2 Colorimetric Measurement Set-up 36 3.3 Preparation of Screen Printed Chips 36 3.4 Methods 39 3.4.1 Surface Preparation 39 3.4.2 Label-free Electrochemical Detection Method 42 3.4.3 Enzyme-based Electrochemical Detection Method 44 3.4.4 Enzyme-based Colorimetric Detection Method 46 4 Results 49 4.1 Optimisation of Detection Methods 49 4.1.1 Preface 49 4.1.2 Optimisation of Surface Preparation 49 4.1.2.1 Preface 49 4.1.2.2 Screen Printing Procedure 50 4.1.2.3 Effect of Pre-treatment Conditions 52 4.1.2.4 Silane Surface Chemistry 54 4.1.2.5 Electrochemical Properties of Inosine Base 57 4.1.2.6 Probe Immobilization 59 4.1.3 Optimisation of Hybridization 61 4.1.3.1 Preface 61 4.1.3.2 Hybridization Time 61 4.1.3.3 Hybridization Temperature 63 4.1.3.4 Optimum Washing Conditions 66 4.1.3.5 Sensitivity of the Detection Methods 68 4.2 Investigation of Optimum Probe Sequences 71 4.3 Determination of Single Base Mutations 74 4.4 Development of Lab-on-a-chip Technology 77 4.4.1 Preface 77 4.4.2 Detection Process in the Cartridge 78 4.4.3 Integrated Process in the Cartridge 80 5 Summary 83 6 References 86 7 Zusammenfassung 93 Introduction 9 1 Introduction Determination of specific nucleic acid sequences in biological and environmental samples can lead to early diagnosis of inherited human diseases as well as identification and detection of pathogens1. The determination of nucleic acids in biological samples consists of three steps: Sample preparation, specific nucleic acid amplification and detection. In the sample preparation step, the extraction and purification of the nucleic acids are performed. First, the relevant cells are lysed by destroying their cellular membrane in order to release the nucleic acids. Then the released nucleic acids are extracted and purified by using different methods. Usually, the amount of extracted nucleic acids is not sufficient for the determination. Therefore, a part of the nucleic acid is amplified, for example, using polymerase chain reaction (PCR). During the PCR, many copies of the specific DNA sequence are created. The reaction is initiated using a pair of short primer sequences which match the ends of the sequence to be copied. Thereafter, each cycle of the reaction copies the sequence between the primers. Primers can bind to the copies as well as the original sequence, so in time the total number of copies increases exponentially. The simplest method for the detection of amplified nucleic acids is gel electrophoresis whereby the DNA is separated according to length and stained with ethidium bromide. However, this method is label intensive and not sequence specific. Sequence specificity can be achieved by transferring the separated DNA to a membrane and hybridising with a radioactively2 labelled probe. This method is very sensitive but complex handling with hazardous radioactive labels are necessary. The other methods which are achieved by labelling the probe with biotin3,4, digoxigenin3,5 or fluorescent dyes6 in order to avoid the use of hazardous radioactive labels are also not suitable for the routine analysis because of the long, expensive and complicated steps of these procedures. Therefore a new, sensitive, low-cost and sequence specific detection of nucleic acid hybridization by using electrochemical DNA biosensors has recently been reported1,8-10 . An electrochemical DNA biosensor is an electrode with immobilised sequence specific single strand DNA (probe) for the identification of target DNA based on its hybridization reaction with its complementary sequence (target) under suitable conditions. The sequence-specific hybridization events can be detected directly (label-free)11-13 or indirectly by using labels 10 Introduction (indicator-based). The labels can be indicators which intercalate into the DNA double helix (metal complexes, antibiotics)14-16 or which interact specifically with guanine bases of DNA10,17-19. The other possible detection method represents the use of substrate which is changed to an electrochemically active end product in the presence of a specific enzyme (enzyme-based)20. Electrochemical DNA biosensors, based on electrochemical transduction of hybridization events, have great promise for the task of pharmaceutical, clinical, environmental and forensic applications. Such devices couple the high specificity of DNA hybridization reactions with the high sensitivity, low cost and portability of electrochemical transducers21. The electrochemical biosensors can be assembled to a miniaturised array22. These miniaturised arrays of DNA biosensors are termed DNA chips. The development of DNA chips is motivated by their potential for application in disease diagnosis, genome sequencing23, the detection of polymorphisms24 and single-base mismatches25. However, such micro fabricated devices are costly and difficult to prepare and handle. For this reason, newly developed DNA chips must offer lower cost and greater material efficiencies to gain acceptance over traditional nucleic acid diagnostic methods. Further reduction in the cost of performing nucleic acid diagnostics can be realized by utulizing less expensive detection methods and electrode materials. The aim of the current work is the development of low-cost DNA chips for the determination of single base mutations, which lead to inherited diseases or disorders (Factor V and Factor II) from blood samples on the fluidic platform in a disposable integrated cartridge in which the processes of sample preparation, PCR and detection are accomplished automatically. The use of a fully automated detection system in combination with its low cost, its simplicity in handling and the disposability of the DNA chips make the determination methods, which are developed for detection of gene-specific inherited diseases, suitable for routine laboratory analysis and point-of-care diagnosis. Theoretical Information 11 2 Theoretical Information 2.1 Electroanalytical Chemistry35,36 2.1.1 Preface Electroanalytical chemistry is concerned with the interplay between electricity and chemistry, namely the measurements of electrical quantities, such as current, potential and charge and their relationship to chemical parameters. The factors effecting the transport of charges across the interfaces between two phases are the important issue in electroanalytical chemistry. One of these two phases contributing to the interface is an electrolyte, through which charge is carried by the movements of ions. Electrolytes can be liquid solutions or fused salts, or they can be ionically conducting solids such as sodium β-alumina, which has mobile sodium ions. The second phase at the interface might be another electrolyte, or it might be a conductor, through which charge is carried by electronic movement. The conductors can be solid or liquid. The transition of the charges, the crossing from one conducting phase into the other at the interface leads to a difference in potential. These two-phase systems can be formed by using two solid (metal-metal) or one solid and one liquid phase (metal-electrolyte). The metal-electrolyte systems describe the basis of electrochemistry whereas the metal-metal systems are used commonly for the measurement of temperature. 2.1.2 Faradaic and Non-faradaic Processes35 Two types of processes occur at the electrodes. One of these is the transferration of charges over the metal-solution interface.
Details
-
File Typepdf
-
Upload Time-
-
Content LanguagesEnglish
-
Upload UserAnonymous/Not logged-in
-
File Pages103 Page
-
File Size-