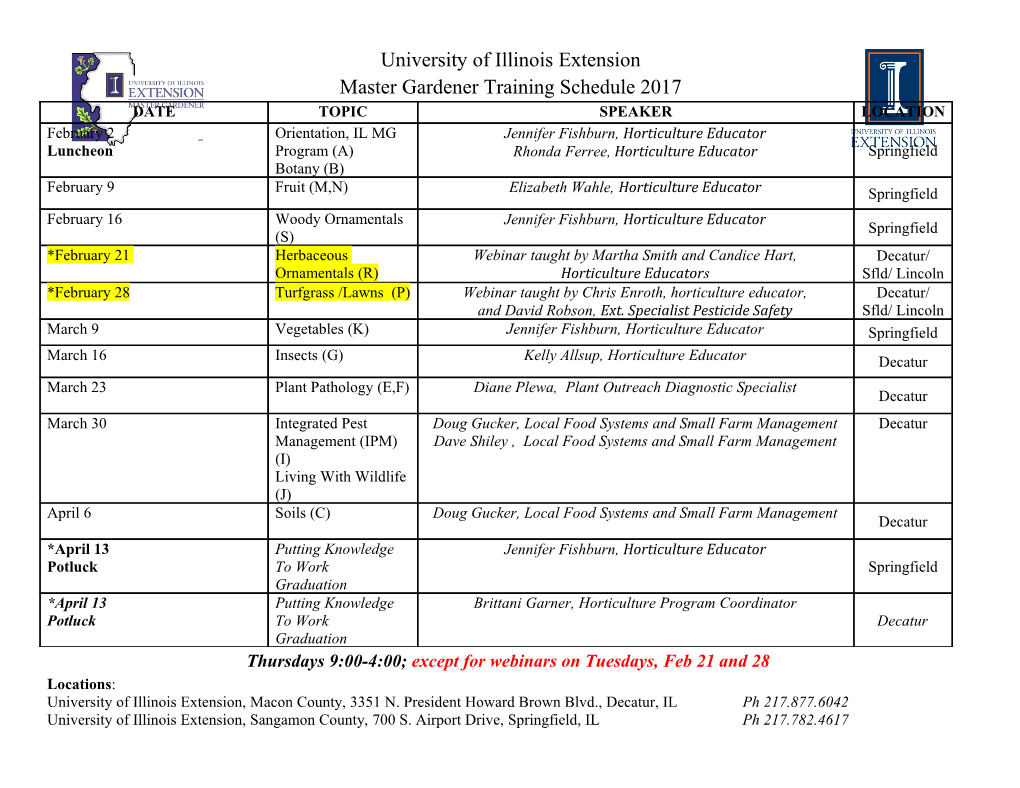
UvA-DARE (Digital Academic Repository) Unfolding plant disease resistance : the involvement of HSP90 and its co- chaperone PP5 in I-2-mediated signalling de la Fuente van Bentem, S. Publication date 2005 Document Version Final published version Link to publication Citation for published version (APA): de la Fuente van Bentem, S. (2005). Unfolding plant disease resistance : the involvement of HSP90 and its co-chaperone PP5 in I-2-mediated signalling. General rights It is not permitted to download or to forward/distribute the text or part of it without the consent of the author(s) and/or copyright holder(s), other than for strictly personal, individual use, unless the work is under an open content license (like Creative Commons). Disclaimer/Complaints regulations If you believe that digital publication of certain material infringes any of your rights or (privacy) interests, please let the Library know, stating your reasons. In case of a legitimate complaint, the Library will make the material inaccessible and/or remove it from the website. Please Ask the Library: https://uba.uva.nl/en/contact, or a letter to: Library of the University of Amsterdam, Secretariat, Singel 425, 1012 WP Amsterdam, The Netherlands. You will be contacted as soon as possible. UvA-DARE is a service provided by the library of the University of Amsterdam (https://dare.uva.nl) Download date:05 Oct 2021 Unfolding plant disease resistance the involvement of HSP90 and its co-chaperone PP5 in I-2-mediated signalling ACADEMISCH PROEFSCHRIFT ter verkrijging van de graad van doctor aan de Universiteit van Amsterdam, op gezag van de Rector Magnificus prof. mr. P.F. van der Heijden ten overstaan van een door het college voor promoties ingestelde commissie in het openbaar te verdedigen in de Aula der Universiteit op vrijdag 11 februari 2005, te 14.00 door Sergio de la Fuente van Bentem geboren te Landsmeer Promotiecommissie Promotor prof. dr. B.J.C. Cornelissen Co-promotor prof. dr. M.A. Haring Faculteit van Natuurkunde, Wiskunde en Informatica Commissieleden prof. dr. T.W.J. Gadella prof. dr. G.C. Angenent dr. K. van Dun dr. M.H. Siderius dr. F.L.W. Takken The research described in this thesis was carried out in the Swammerdam Institute for Life Sciences, Plant Pathology department, University of Amsterdam, Kruislaan 318, 1098 SM, Amsterdam, The Netherlands aan mijn ouders en broers voor Katinoula Front cover: microscopical image of the localisation of a yellow fluorescent fusion protein in a cowpea (Vigna unguiculata) protoplast (see chapter 2) Voorzijde: microscopische opname van de localisatie van een geel fluorescerend fusie-eiwit in een kousenband (Vigna unguiculata) protoplast (zie hoofdstuk 2) Back cover: localisation of yellow and cyan fluorescent fusion proteins in cowpea (Vigna unguiculata) protoplasts (see chapter 2) Achterzijde: localisatie van geel en cyaan fluorescerende fusie-eiwitten in kousenband (Vigna unguiculata) protoplasten (zie hoofdstuk 2) Printing of this thesis was financially supported by the J.E. Jurriaanse fonds and De Ruiter Seeds Contents Page Chapter 1 General Introduction 7 Chapter 2 The subcellular localisation of plant protein Ser/Thr 33 phosphatase 5 isoforms is determined by alternative splicing Chapter 3 Protein Ser/Thr phosphatase 5 interacts with 53 multiple disease resistance proteins but is not essential for their function Chapter 4 The I-2 disease resistance protein and protein Ser/Thr 73 phosphatase 5 interact with heat shock protein 90 Chapter 5 General Discussion 93 Samenvatting 110 Dankwoord 112 Color figures 114, 118 Chapter 1 General Introduction Plant pathogens can cause epidemics that have a major impact on agriculture and even society as a whole. For instance, the outbreak of potato late blight (causal agent: Phytophthora infestans) led to the Irish potato famine in the 1840s, during which millions of people died of starvation. Traditional selection of crop varieties with high yields instead of disease-resistant varieties has rendered them vulnerable to pests. Each year, diseases lead to losses of up to 15% worldwide, costing many billions of euros. Breeders have started to cross disease resistance traits from wild relatives into their crops since the 1930s. Unfortunately, breeding is time-consuming and often resistances are overcome by pathogens within years. Therefore, this method is too slow to keep up with the development of new diseases. As an additional method, diseases are combatted by the use of fungicides and pesticides, carrying with it many risks for the environment. In order to achieve sustainable agriculture, research has to be done to uncover the mechanisms behind disease resistance in plants. The elucidation of the molecular processes underlying plant defence against pathogens may provide leads to improve crop resistance in the future. In the first section of this general introduction, several aspects of plant disease resistance genes are decribed. Chapters 2-4 of this thesis show the characterisation of protein Ser/Thr phosphatase 5 (PP5) in plants and the identification of PP5 and heat shock protein 90 (HSP90) as interactors of the I-2 disease resistance gene product. For easier understanding of those chapters background information is given related to PP5 and HSP90 in the second and third section of this General Introduction, respectively. Chapter 1 Plant disease resistance (R) genes Types of resistance Most plants are resistant to most pathogens, indicating that susceptibility is relatively exceptional in nature. The most abundant type of resistance is so-called non-host resistance, i.e. all genotypes of a species are resistant to all genotypes of a particular pathogen. This implicates that the plant either expresses effective basal defence or that the pathogen lacks adequate tools to invade this specific plant species. The genetic basis for non-host resistance is largely unknown. Variaties of a plant species can also express partial resistance to all different genotypes of a pathogen. This horizontal resistance is often conferred by multiple genes and thus difficult to breed. However, resistance of a certain plant variety can be restricted to specific races of a particular pathogen only, whereas other races cause disease on this plant genotype. This race-specific or vertical resistance is mono- or oligogenic and therefore easier to breed into crops. Based on studies on the genetic interaction between different genotypes of flax (Linum usitatissimum) and races of the flax rust fungus (Melampsora lini), Flor (1942) formulated the gene-for-gene hypothesis to explain race-specific disease resistance. This genetic hypothesis states that in the plant dominant disease resistance (R) genes are present that match dominant, race-specific avirulence (Avr) genes in the pathogen; when a pathogen carrying the Avr gene infests a plant containing the matching R gene, the plant is able to recognise the pathogen and triggers an effective defence response. Later, a biochemical interpretation of the gene-for-gene hypothesis was proposed that suggested that R genes encode receptors for Avr-encoded proteins. The receptor binds the Avr protein and subsequently triggers a signalling cascade leading to disease resistance. Later studies, however, have revealed that a direct interaction between R proteins and Avr proteins is rather the exception than the rule (see below). R protein structure Based on their predicted structural features, most R gene products fall into three main classes (Figure 1; Table 1; Dangl and Jones, 2001; Martin et al., 2003). Class 1 is the largest class and consists of R proteins with a central nucleotide binding (NB) domain with characteristics shared between Apaf-1, R proteins and CED-4 (ARC), and a C-terminal leucine-rich repeat (LRR) domain. Apaf-1 and CED-4 are animal proteins involved in apoptosis, a type of programmed cell death. Interestingly, defence responses activated by plant R proteins often results in the hypersensitive response (HR), which is a specific form of programmed cell death. The central NB-ARC domain of the R proteins I-2 and Mi-1 specifically bind and 8 Introduction hydrolyse ATP in vitro (Tameling et al., 2002). At their N-termini, R proteins of this class either contain a coiled-coil (CC) domain or a TIR (homology with animal Toll and Interleukin-1 receptors) domain (Table 1; Martin et al., 2003). CC-NB-ARC-LRR and TIR- NB-ARC-LRR proteins will be further referred to as CNL and TNL proteins, respectively. C/TNL proteins are predicted to be cytosolic proteins, of which some are attached to the cytoplasmic side of the plasma membrane (Boyes et al., 1998), while others localise in the nucleus (Deslandes et al., 2003). Strikingly, many animal species contain NB-ARC-LRR proteins termed nucleotide-binding oligomerisation domain (NOD) proteins (Figure 1). NODs are involved in innate immunity, which contrasts with adaptive, antibody-based immunity in mammals. This suggests a common mechanism for NB-ARC-LRR proteins in terms of activation of defence responses in animals and plants. Indeed, a plant R protein has been shown to oligomerise after its activation (P. Mestre, Poster 069, EPSO congress 2004), a feature that is common to NOD proteins, and the NB-ARC proteins Apaf-1 and CED-4 as well. Figure 1 (see page 114 for color version of this figure). Proteins involved in innate immunity in plants, flies and mammals are structurally conserved. Representative members of protein classes involved in disease resistance in plants, flies and mammals. Abbreviations: CC, coiled-coil;
Details
-
File Typepdf
-
Upload Time-
-
Content LanguagesEnglish
-
Upload UserAnonymous/Not logged-in
-
File Pages118 Page
-
File Size-