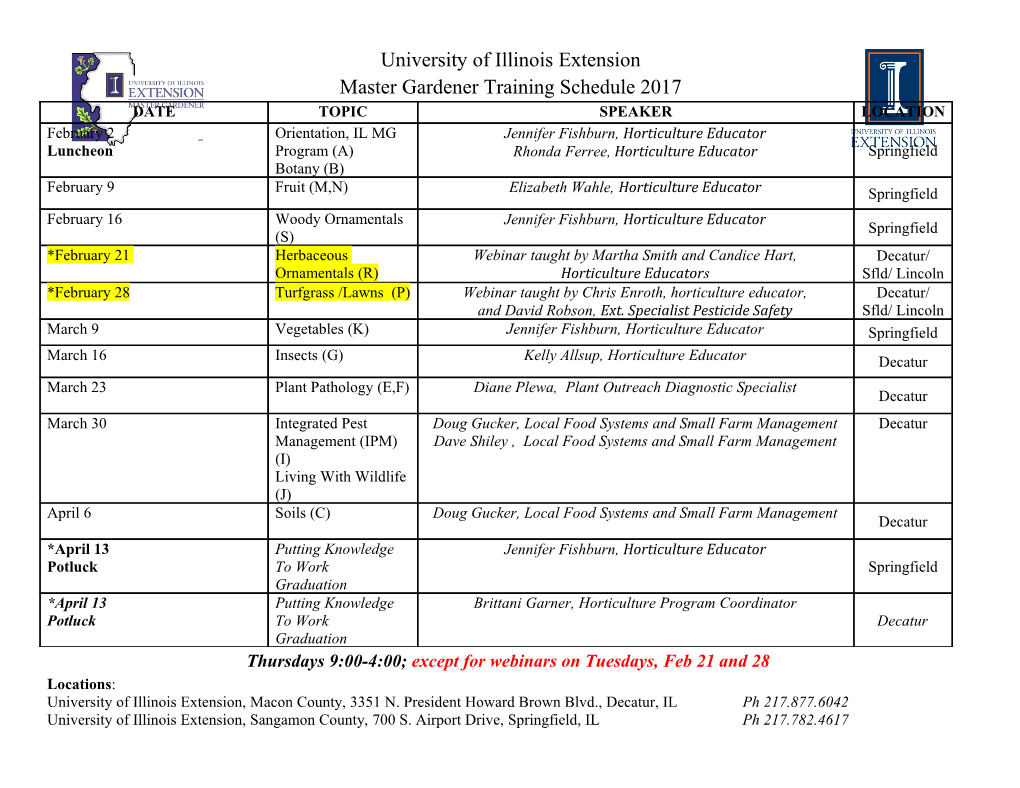
APPENDIX I Methods used in studying modern pyroclastic deposits 1.1 Physical analysis The methods used to measure these, and the major uses of these measurements are set out below. Geologists who work solely on ancient volcanic rocks often have only a limited conception of the techniques 1.1.1 THICKNESS employed to study Recent unconsolidated pyroclastic deposits, and so may not fully understand how the data Maximum thickness of a pyroclastic fall deposit is are obtained or expressed. Many of the problems measured in centimetres or metres, and the measurements encountered in the study of modern pyroclastic deposits are used to construct an isopach map. In the figures are similar to those found in sedimentary rocks, where accompanying Chapter 6 there are a number of examples grainsize, grain shape, geometry of the deposit and of such maps, which in many cases, are a meaningful internal fabric must be the tools used to determine the indication of: physical processes controlling their formation and de­ (a) the vent position, position. The pioneers in this type of approach were (b) the dispersal, which can be related to the type of undoubtedly Japanese volcanologists (e.g. Kuno 1941, eruption, and Aramaki 1956, Katsui 1959, Murai 1961, Kuno et al. (c) the volume of the deposit. 1964), whereas G. P. L. Walker (e.g. 1971, 1973b) can be credited with extending and developing the approach. The following are properties that are now routinely Construction of an isopach map entails mapping out measured in the physical analysis of modern pyroclastic the deposit, sometimes over large areas. However, by deposits: mapping we do not mean tracing lithological boundaries between deposits, as these are usually so complex that no thickness attempt is made to draw them. Thus, a map of a Recent maximum grain size pyroclastic fall deposit generally shows its inferred grainsize distribution original distribution, and not its present outcrop pattern, proportions of components as between datum points the deposit could be partially or crystal content of pumice clasts completely eroded. Indeed, non-welded pyroclastic density and porosity deposits may be ephemeral (Ch. 10). In addition, outcrops may be so rapidly overgrown and badly 469 470 APPENDIX I: STUDYING MODERN DEPOSITS weathered that they cannot be used. For example, new 1.l.2 MAXIMUMGRAINSIZE roadcuts in the tropical Caribbean are sometimes completely overgrown within four or five years. Because Measuring the average maximum juvenile and lithic clast pyroclastic fall deposits can change markedly laterally, it size is an important field technique, which involves is preferable to use reasonably closely spaced datum measuring, at numerous localities, the long axis of several points. Although spacing ultimately depends on the type of the largest clasts in a deposit. In some detailed sections of eruption, for the large plinian fall deposits localities the variation in grainsize between different layers of one within 1 km of each other are favoured. Within these deposit is measured. Usually the sizes of the three or five distances correlation is more certain, and internal largest clasts are then averaged, and this would closely changes can be carefully documented. This is very approximate the coarsest one-percentile often quoted by important in correlating deposits between localities and sedimentologists. Average maximum pumice (or scoria) in understanding the eruption and its stratigraphy. and lithic sizes can be plotted up as isopleth maps (Chs The volumes of air-fall deposits have been calculated 6-8). As with isopach maps, maximum-size isopleth from isopach maps in various ways. A common method maps are important in locating the vent from which involves measuring the area enclosed by each isopach and pyroclastic fall deposits were erupted, and for comparing then to plot area against thickness on a log-log 'area plot' their dispersal in order to characterise the type of (Fig. 6.18). A curve, or two straight lines (Rose et al. eruption. However, for pyroclastic fall deposits, such 1973), are fitted to the data, and integration of this curve isopleth maps have certain advantages over an isopach gives the volume. Other methods include plots of volume map, because at some localities it may not be the original against thickness and plots based on theoretical isopach depositional thickness that is being measured. The top of shape (Froggatt 1982). All of these methods involve a deposit may have been eroded by either a later surge or extrapolation of isopachs to the low-thickness distal limits flow, which is sometimes common with near-vent plinian of the deposit where outcrop may be poorly eroded. This deposits, or by later local erosion or soil-forming introduces major uncertainty for larger, more widely processes. It may also have been overthickened by dispersed types of deposits, especially where secondary secondary slumping, especially if the fall was deposited thickening might have been important (Ch. 6). To try to on a steep slope. Also, some extremely widely dispersed resolve this, G. P. L. Walker (1980, 1981b, c) developed (ultraplinian) deposits may be thickest just down-wind of an independent method for estimating the total volume of the vent (Chs 6 & 8), and secondary thickening of distal plinian deposits, based on crystal concentration studies ash may occur (Ch. 6). (see below) of the Taupo ultraplinian, and Waimihia and Measurements of maximum clast size are also used to Hatepe plinian deposits. Once the total volume erupted analyse the energetics of pyroclastic fall eruptions. This is had been estimated from the proportion of free crystals especially so for large ballistic clasts; that is, those clasts relative to the magmatic ratio as represented in pumice which are so heavy that they follow ballistic trajectories clasts, a straight-line extrapolation could be made at the and are unaffected by wind drift. The distance at which low thickness end on an area plot to a selected limiting ballistics fall from the vent (that is, their range) can be thickness value, so giving the same volume as would be used to estimate initial gas thrust velocities from the vent, calculated by integration of the area curve. It was found or the muzzle velocities of the ballistics from the vent that for all three deposits extrapolations to the same lower (Ch. 6). L. Wilson (1972) presented tables of calculated limiting thickness of 1 Ilm had nearly identical slopes. ranges for particles of varying radii and density, launched Total erupted volumes of other plinian deposits can be at speeds between 10 and 1000 m S-l and various conveniently estimated on an area plot by extrapolation angular elevations. These are reproduced in Table 1.1, parallel to this slope, using the same limiting thickness of and cover most ballistic clast sizes (Ch. 6). 1 Ilm (G. P. L. Walker 1981b; Table 6.2). For plinian deposits the muzzle velocity can be Measurements of the variation in the thickness of conveniently calculated from measurements of maximum pyroclastic flow and surge deposits are less meaningful in clast size using the equation of L. Wilson (1976, 1978): terms of an indicator of vent location. This is because (8grooo) both are gravity-controlled mass flows, which therefore uij= (Ll) tend to pond in depressions. However, thickness is 3CQo important in calculation of the volumes of such deposits. where Uo is the velocity of the gas (or muzzle velocity), C is the drag coefficient (-1 for plinian velocities), Qo is the PHYSICAL ANALYSIS 471 Table 1.1 Logarithms (base 10) of the ranges of larger pyroclastic particles (after L. Wilson 1972). Velocity Density Launched at 45° radius Launched at 66° radius Launched at 37° radius (m S~l) (g cm-3) (cm) (cm) (cm) 1.0 3.0 10.0 30.0 100.0 1.0 3.0 10.0 30.0 100.0 1.0 3.0 10.0 30.0 100.0 3.5 2.9556 3.0032 30075 2.8261 2.8736 2.8786 1.9790 20211 2.0268 2.5 2.9369 3.0017 3.0070 2.8073 2.8719 2.8785 1.9614 2.0207 2.0267 10 1.0 2.8559 2.9914 30060 2.7242 2.8601 2.8770 1.8830 2.0099 2.0254 0.5 2.7579 2.9760 30043 2.6222 2.8427 2.8753 1.7846 1.9937 2.0236 3.5 3.6440 3.9315 3.9583 3.4896 3.8010 3.8290 2.6641 2.9505 2.9775 2.5 3.5719 3.9208 3.9571 3.4235 3.7897 3.8276 2.5897 2.9395 2.9762 30 1.0 3.3383 3.8680 3.9483 3.1834 3.7329 3.8180 2.3497 2.8843 2.9673 0.5 3.1364 3.7965 3.9356 2.9752 3.6544 3.8036 2.1373 2.8070 2.9540 3.5 4.0418 4.6867 4.9660 3.8644 4.5529 4.8358 3.0228 3.7156 3.9873 2.5 3.7372 4.6173 4.9514 3.7468 4.4821 4.8195 2.9034 3.6437 3.9721 100 1.0 3.6042 4.3999 4.8817 3.4194 4.2548 4.7461 2.5717 3.4116 3.9026 0.5 3.3549 4.2139 4.7912 3.1673 4.0556 4.6504 2.4068 3.2078 3.8097 3.5 4.23894.7075 5.0257 5.3861 5.6581 4.04104.5111 4.86735.23575.5274 3.1877 3.6669 4.0293 4.3988 4.6906 2.5 4.11104.58494.9177 5.2957 5.5914 3.91114.38524.75735.13685.4571 3.05683.53943.91574.30004.6212 300 1.0 3.7592 4.2319 4.6183 5.0127 5.3732 3.5575 4.0304 4.4499 4.8381 5.2261 2.69973.17703.59934.00064.3900 0.5 3.4931 3.9647 4.3891 4.7744 5.1779 3.29063.75904.21034.59205.0199 2.43022.90153.35493.75214.1815 3.5 4.29594.77445.13235.54045.9162 4.0926 4.5719 4.9631 5.3820 5.7887 3.2356 3.7238 4.1204 4.5432 4.9575 2.5 4.1652 4.6458 5.0139 5.4302 5.8157 3.9602 4.4406 4.8427 5.2624 5.6795 3.10243.59123.99644.42274.8464 600 1.0 3.8073 4.2817 4.6933 5.1087 5.5245 3.60134.07584.51554.92565.3690 2.74043.2191 3.66074.08364.5308 0.5 3.5373 4.0098 4.4526 4.8524 5.2928 3.3309 3.8005 4.2660 4.6621 5.1253 2.4679 2.9400 3.4069 3.8174 4.2831 3.5 4.34264.82755.21125.65106.1029 4.1354 4.6207 5.0348 5.4885 5.9963 3.27553.76934.18814.64825.1767 2.5 4.21004.6948 5.0861 5.5273 5.9760 4.0014 4.4857 4.9075 5.3542 5.8474 3.1407 3.6332 4.0573 4.5119 5.0191 1000 1.0 3.8477 4.32314.75175.18105.6329 3.63844.11394.56704.9921 5.4732 2.7752 3.2547 3.7089 4.1463 4.6333 0.5 3.5749 4.0479 4.5032 4.9130 5.3772 3.36543.83584.31084.71735.2033 2.5003 2.9732 3.4489 3.8687 4.3578 effective density of the volcanic gas in the vent I.1.3 GRAINSIZE DISTRIBUTION (0.25 kg m-3 for dusty gas), g is the acceleration due to gravity, ro is the radius of the average maximum clast at Mechanical or granulometric analyses are used as the vent and 00 is its density (generally taken as 2.5 g cm-3 main source of data when examining the grainsize for lithics).
Details
-
File Typepdf
-
Upload Time-
-
Content LanguagesEnglish
-
Upload UserAnonymous/Not logged-in
-
File Pages59 Page
-
File Size-