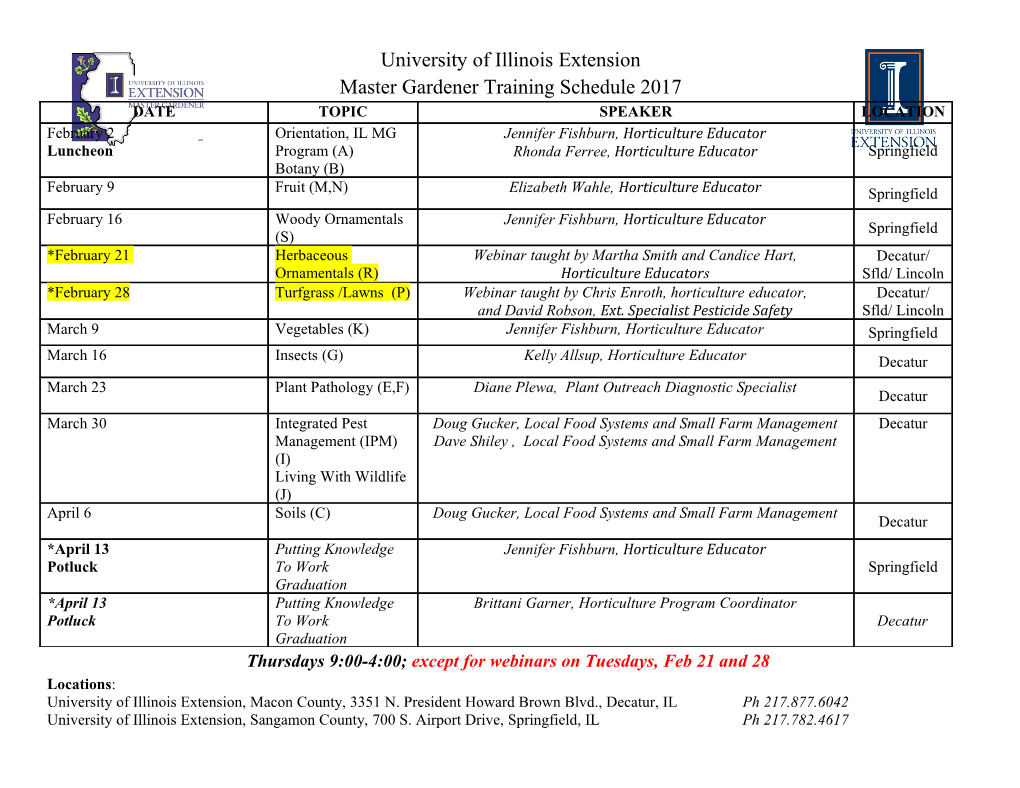
Western Australian School of Mines Department of Exploration Geophysics Gamma Ray Spectroscopy for Logging While Drilling in Mineral Exploration Ida Hooshyari Far This thesis is presented for the Degree of Doctor of Philosophy of Curtin University March 2018 I Declaration “To the best of my knowledge and belief this thesis contains no material previously published by any other person except where due acknowledgment has been made. This thesis contains no material which has been accepted for the award of any other degree or diploma in any university.” Signature: Date: 19/03/2018 II To my dear mother, for all her love and all her tough love To my great father, for his love, support and his dedication to our family To my life and love partner, for his love, care and being an amazing being III Thesis Abstract Gamma ray spectroscopy has been in use in the mineral exploration industry for decades, with much pioneering work done in the 1970’s to 1980’s, yet many of the techniques used to process the recorded spectrum have not improved significantly in the last 30 years. Technological developments in slim logging tools appear to have not kept pace with other technological developments, such as advances in scintillator materials for better gamma detectors, and neutron generators for electronic control of radiation. Yet the ability to collect good quality gamma-ray energy spectra with 256 or more channels is common and the computational resources for better data processing are now abundant. Despite such advances there hasn’t been much change in the way natural gamma spectra are analysed, in the borehole or in airborne radiometrics, except for noise reduction techniques such as Noise Adjusted Singular Value Decomposition (NASVD) and Maximum Noise Fraction (MNF). Natural gamma-ray logs currently estimate concentrations of natural radioisotopes of uranium, thorium and potassium (U, Th and K) based on analysis of spectral peaks in the gamma-energy spectrum and estimation the decay series is in radioactive equilibrium. However, there is, potentially, other useful lithological information hidden within the recorded gamma-ray spectrum. This extra information lies in the gamma rays scattered by the rock, normally rejected as noise or background to spectral peaks. These gamma rays are the ones that have interacted with elements other than K, U, and Th and provide new physical information about the rock matrix. The research in this thesis principally explores the use of a ratio between photoelectric absorption and Compton scattering and uses a calculation of a ratio (the Heavy Mineral Indicator) of these effects to measure changes in effective atomic number (Zeff) in the formation. Furthermore, it is postulated that as the relative atomic number of iron is greatest amongst the most common crustal elements then the Heavy Mineral Indicator (HMI) is generally an indication of the iron content of the rock in most cases. Simulation, laboratory scale models, and field experiments all demonstrate that the natural gamma-ray spectrum recorded during borehole drilling (Logging-While- Drilling) can be used to track changes in heavy element concentrations with HMI, and HMI correlates well with iron-rich zones. The best regions within the gamma spectrum to be exploited are energies between 50-150 keV and between 200-500 I keV; the ratio of gamma-ray counts in these energy windows provides a good measure of Zeff variation. It was found that the small gamma-ray detectors used in wireline logging collected too few gamma rays to be useful in measuring changes in Zeff with HMI. Wireline logging speeds are too fast (even at 2 m/min) to collect sufficient scattered gamma rays to produce statistically useful results. Thus, it is concluded that logging-while–drilling measurement is the only viable means to detect changes in Zeff because logging speed is much slower. Another novel aspect of looking at the relationship between HMI and iron is the application of this type of analysis to conventional litho-density tools that use an isotope gamma-ray source. Data were analysed from experimental litho-density tools designed to measure density in iron ore deposits in the Pilbara area of Western Australia. The litho-density gamma spectra from iron ore formations provided detectably higher HMI measures as expected due to relatively high Zeff in this environment. Thus, HMI values can act as a proxy for an iron assay to differentiate iron ore from waste rock in near real-time. An opportunity to test HMI measurements on airborne radiometrics represented a slight side-track in the research. Advances in airborne gamma ray spectrometers and the implementation of low-level surveys provide high quality spectra similar to logging while drilling approaches. The analysis of a well-studied radiometrics data set from Elashgin, Western Australia, show very clearly that iron- rich soils and surface formations are mapped with HMI. Thus, using the same approach in analysing natural gamma rays from boreholes allows maps of Fe and K, U, Th to be made simply by reprocessing legacy data. This work has demonstrated that analysing the scattered gamma rays in the earth may be used to see changes in soils and rocks non-invasively using natural gamma radiation. Additionally, the measure of these changes, the Heavy Mineral Indicator, is generally related to the iron content of the material. In combination, these two findings alone represent a significant advance in how we may use spectral- gamma measurements in the earth sciences. II Acknowledgments Firstly, I would like to thank sincerely my supervisor Anton Kepic for his guidance and his support. It has been a great privilege to work with him and to benefit from his brilliant ideas. I am also very grateful to my co-supervisor Michael Carson for his assistance, enthusiasm and great support. I am also thankful to Anousha Hashemi from BHP Billiton for providing me her time and knowledge. I would like to acknowledge the Deep Exploration Technology Commonwealth Research Centre (DETCRC) for their financial assistance. Additionally I wish to acknowledge Curtin University for my CIPRS scholarship. Last but not least, I thank my family in Iran for supporting me and giving me so much positive energy despite the distance and my beautiful friends in Australia. III List of publications Extended abstracts Hooshyari, I., A. Kepic, M, Carson, 2016, Gamma-ray spectra analysis for Lithology investigation. Near Surface Geoscience 2016 - First Conference on Geophysics for Mineral Exploration and Mining, 4-8 September 2016. Barcelona, Spain: EAGE. Hooshyari, I., A. Kepic, A. Greenwood, A. Podolska, 2015, A New Logging While Drilling Approach for Natural Gamma Spectroscopy with Diamond Drilling. Joint assembly, AGU-GAC-MAC-CGU, 3-7May 2015, Montreal, Canada Hooshyari-Far, I., A. Kepic, and A. Podolska. 2015. A new approach provides opportunities for spectral gamma analysis in boreholes for mineral exploration. ASEG Extended Abstracts 2015: 24th International Geophysical Conference and Exhibition. 1-4 February 2015, Perth, Australia Hooshyari, I., Kepic, A., Javadipour, Sh., 2014. Resistivity and Induced polarization technique for mapping hematite rich areas in Iran: ASEG Conference 2015 Kepic, A., A. Podolska, I. Hooshari-Far, and A. Greenwood, 2015, New scintillators and method for spectral gamma logging in slim boreholes. Near-Surface Asia Pacific Conference, Waikoloa, Hawaii, 7-10 July 2015. 220-223. Journal articles in preparation Hooshyari, I., Kepic, A., Carson, M, Heavy metal indicator in logging while drilling, (Geophysics) Hooshyari, I., Kepic, A., Carson, M, A novel approach in analysing full spectrum airborne radiometric data (Geophysics Research Letters) IV Contents Declaration ................................................................................................... II Thesis Abstract .............................................................................................. I Acknowledgments ....................................................................................... III List of publications...................................................................................... IV List of Figures ............................................................................................. IX Nomenclature ........................................................................................ XVIII Chapter 1. Introduction .................................................................................1 1.1 Motivation .....................................................................................1 1.2 Research aims ................................................................................3 1.3 Thesis outline ................................................................................4 Chapter 2. Introduction to gamma-ray spectroscopy ...................................6 2.1 Nuclear physics for logging application .........................................6 2.1.1 The origin of natural gamma rays............................................6 2.1.2 Radioactivity of the elements in rocks .....................................6 2.1.3 Gamma-ray attenuation ...........................................................7 2.1.4 Fundamentals of gamma-ray interactions ................................9 2.1.5 Gamma-ray spectroscopy ...................................................... 13 2.1.6 Gamma-ray measurement application ................................... 14 2.1.7 Gamma-ray measurement instrumentation ...........................
Details
-
File Typepdf
-
Upload Time-
-
Content LanguagesEnglish
-
Upload UserAnonymous/Not logged-in
-
File Pages170 Page
-
File Size-