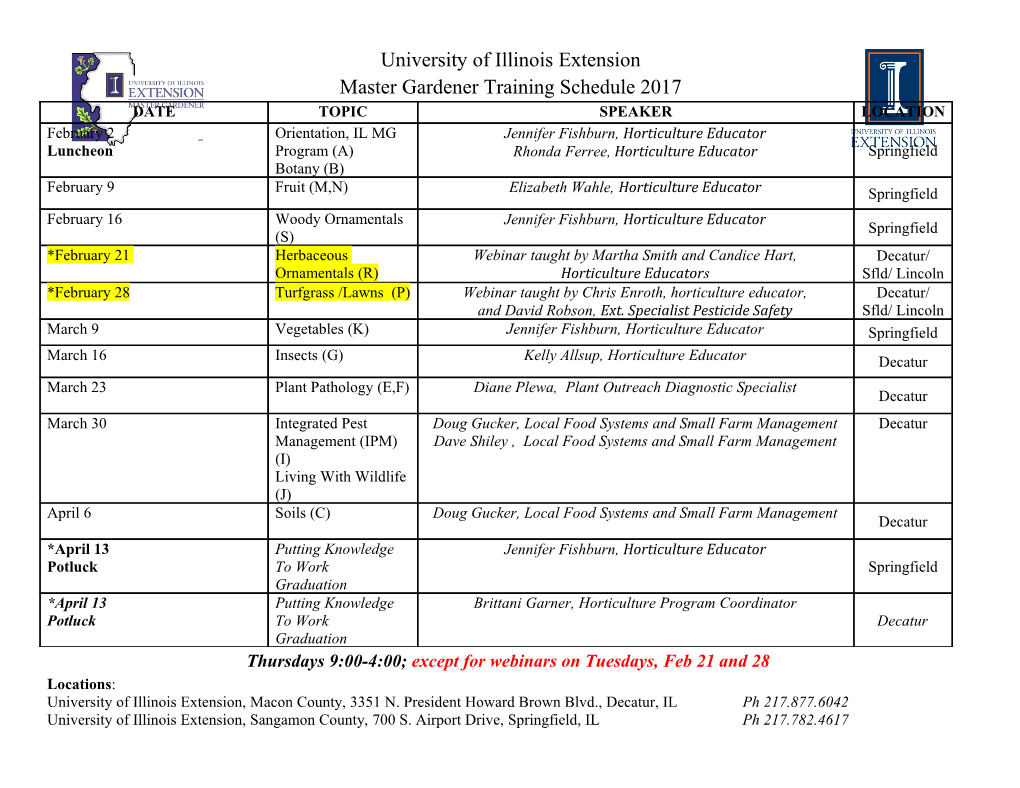
ISIJ International. Vol. 36 (1 996), No, 12, pp. 1423-1438 Revie w High-Strain-Rate Superplasticity in Metallic Materials and the Potential for Ceramic Materials Kenji HIGASHl.MamoruMABUCH11)and Terence G. LANGDON2) Department of Mechanical Systems Engineering, College of Engineering. Osaka Prefecture University. Gakuen-cho. Sakai. Osaka-fu, 593 Japan. 1) National Industrial Research Institute of Nagoya. Hirate-cho. Kita-ku, Nagoya. Aichi-ken, 462 Japan. 2) Departments of Materials Science and Mechanical Engineering. University of Southern California, Los Angeles, CA90089-1453, USA, (Received on June 10. 1996, accepted in final form on September9. 1996) High-strain-rate superplasticity (i.e,, superplastic behavior at strain rates over IO' s~ ') has been observed in manymetallic materials such as aluminum alloys and their matrix composites and it is associated with an ultra-fine grained structure of less than about 3/sm. Its deformation mechanismappears to be different from that in conventional superplastic materials. Experimental investigations showedthat a maximum elongation was attained at a temperature close to the partial melting temperature in manysuperplastic materials exhibiting high-strain-rate superp]asticity. Recent]y, a newmodel, which wasconsidered from the viewpoint of the accommodationmechanismby an accommodationhelper such as a liquid or glassy phase, wasproposed in which superplasticity wascriticaliy contro[led by the accommodationhelper both to relax the stress concentration resulting from the sliding at grain boundaries and/or interfaces and to limit the build up of internal cavitation and subsequent failure. Thecritical conditions of the quantity and distribution of a iiquid phase for optimizing superplastic deformation wasdiscussed and then applied to consider the possibility of attaining high-strain-rate superplasticity in ceramic materials. KEYWORDS:grain boundary sliding; accommodation helper, fine grain size; cavitation; Iiquid and amorphousphases. typical forming rate used for the conventional super- 1. Introduction plastic materials, but rather close to the commercial hot Polycrystalline materials, when pulled in tension, working rates of lO1 to 102 s~1. The high-strain-rate generally break after rather modest amounts of plastic superplasticity phenomenonwas originally observed in deformation, about less than 500/0 in elongation. Under 1984 in a metal-matrix composlte,6) then found in 1985 superplastic conditions of tensile testing, however, very in a mechanically-alloyed material2) as well as metallic high elongations of morethan 500 o/o or in a specific case alloys produced by moreconventional methods.29) Since over 5OOOo/o can be obtained. Superpiasticity is defined 1990, the high-strain-rate superplasticity phenomenon as the ability of a polycrystalline materials to exhibit, in has been studied extensively in somedetail, in metal- composites,7 ~27) jn a generally isotropic manner, very high tensile elonga- matrix mechanically alloyed materi- tions prior to failure. 1) Usually superplasticity is attained als,5) and in moreconventional alloys.30.31) in the low strain rate range from l0~5 to lO3 s~ 1. The Technologically, high-strain-rate superplasticity is of superplastic strain rate range is rather low for commer- great interest because it is expected to result in eco- clal forming of structural materials and therefore the nomically-viable, near-net-shape forming techniques for commercial viability of superplastic materials is limited. the automobile, aerospace, and even semi-conductor In recent years, however, research into superplasticity industries. Therefore, in order to focus on the factors has developed substantially, to the extent that there are contributing to the attainment of superplasticity at high now several important directions for future investiga- strain rates and to discuss the deformation mechanisms tions. Onenewarea is superplastic behavior at relatively in high-strain-rate superplastic materials, workshopson high strain rates over l0~2 s~ I in metallic alloys2 ~21) in- high-strain-rate superplastic forming were held twice at cluding metal matrix composites,6~21) which are pro- LawrenceLivermore National Laboratory on March15, 1994,32) duced by a combination of powder metallurgy and/or and at OsakaPrefecture University on May22, 1995,33) some advanced processing methods.22~27) This high- as shown in Figs. I and 2. Clearly from the strain-rate superplasticity is defined from JIS H7007 by drawings indicating the relationship betweenelongation strain for JapaneseStandards Association as superplasticity which and rate several aluminumalloys with various appears whenthe rate of strain is l0~2s~1 or over.28) grain sizes produced by different processing routes (Fig. 1) size These strain rates are considerably higher than the and a graln dependenceof the superplastic strain 1423 @1996 ISIJ t ISIJ Inte~national, Vol. 36 (1 996), No. 12 1ooOO cavitation, It will be proposed that the dominant de- Al AIloys formation mechanismfor al] the high-strain-rate [~~~ ~~~] super- 'e 2oeo, 500'c plastic materials is grain sliding, special ~~l supral' 450'c// boundary and a AI 7475, 504'c ~~l ~~.' 1000 Ai 21 24-0,e~1~zr' 47s'c accommodationprocess by an accommodationhelper ,: llquid is for o such as a phase suggested to be responsible ,p in fine a, the observed hlgh-strain-rate superplasticity very t: o grained materials. This is requlred only to attain UJ [~] not an IOO AI 21 24-20e/~Si d~ 4 IN 902i &9021 ultra-fine grain size, but also to relax the stress 475'C concen- ~~] trations at triple junctions and around the hard particles 'U 21 24~O~~~ilJ'T]1$SiCw 52s'c ' such as relnforcements in the meta] matrix composites 10 in d3 .2 J1 O1 02 order to attain superplasticity at very high strain ld 5 104 1 10 1 1Oo I 1 103 rates. Strain Rate (~~1 ) Further consideratlon for a special process by an ac- Fig. l. Thedrawing indicating high-strain-rate superplasticity commodationhelper such as a liquid phase is discussed, In aluminum alloys with various grain sizes, taken and will include a quantitative estimation for the opti- from the front of the final report of the first page volume and distribution of a liquid phase to relax workshopon high strain rate superplastic forming. 32) mum the stress concentrations during high-strain-rate super- plastic fiow. Finally, this review will give guidelines for 104 Consolldated Alloys from the future research and development of materials with A Amorphousor Nanocrystalline capabilities for high-strain-rate superplastic forming 103 Powders Al•Ni•Mm•Zr D Physlcal VaporDeposltlon operations such as metallic alloys and composites, as Mechanlcally Alloyed well as intermetallics or ceramics. 102 @AlumlnumAlloys IN9021 IN905XL U, 2. Microstructural Features in High-strain-rate Super- 101 •CJO • IN9052 plastic Metallic Materials SeN4p'AI•Zn•Mg-CuO CSICp/lN9021 O SeN4p/Al•Mg•SIO IVQAl•Cr-Fe ,11 100 p!Al-Zn•Mg of the superplastic properties of S~N4 O/ Al-N i•Mm•Zr Asummary a number S~N4p!Al•Mg Al•Nl-Mm = Os~N4p/Al-Cu-Mg of advancedstructural materials is reviewed in Table 1. CD i3N4w/AI-Zn-Mog 1o'1 S (C)~MAI-Zn-Mg-Cu•Zr The materials include mechanically alloyed materials, U, ~rv~*,PM Al-Cu•Zr O PMAl-Mg-Zr consolidated alloys from amorphouspowders O SI3N4w/Al-Cu•M SieN4p/AI-Cu-Mg or nano- u) •2 crystalline alloy, ,:; 1o powders, a vapor quenched metal ,:L MAl-Mg-Mn matrix composites, as well as powder (PM) and ingot ,D 1o'3 (IM) metallurgically processed alloys. Top represents the a= Cl) PowderMetallurglcally superplastic Ti the incipient IQ' Processed Alloys optimum temperature, •4 point, T~ the solidus the 1o Ingot Metallurgically meitlng temperature and d grain l~ processed Alloys is size. It noted that all the materials have very fine 1o•5 grained structures from O.5 to 3pmin size, except IM o.ol 0.1 1 10 loo 7475 which has a coarse grain size of about 20,am. The '1/um'l Inverse graln size, d deformation behavior of a material at elevated tem- Fig. 2. The drawing Indicating a relationship between the peratures can be represented by a constitutive equation strain size in superplastic superplastic r'ate and grain which incorporates an activation energy term as given aluminumalloys, taken from the front pageof the final by34'35) pamphlet of the second workshop on high-strain-rate superplastic formlng.33' ._AkGTb(~)P( (T G(T n -Q o Doexp (1) it is RT rate in manyaluminumalloys (Fig. 2), nowwidely is is is is acceptable that high-strain-rate superplasticity asso- where i the strain rate, h the Burgers vector, (T ciated with a very small grain size. It appears, however, the flow stress, (ro is the threshold stress, Gis the shear that the relationshlps between the microstructure and modulus, Do is the pre-exponential factor for diffusion, the mechanical properties of the materials exhibiting Qis the activation energy for superplastic fiow (presum- high-strain-rate superplastlcity are not fully understood. ably Qs~pe'plasti.=Qdirr~sion)' R is the gas constant, T is Currently, the origin of hlgh-strain-rate superplasticity the temperature in degrees Kelvin, n is the stress exponent is under considerable discussion. at typically 2 for superplastic fiow, p is the grain size The first purpose of the present review is to explore exponent at 2-3 depending on the dominant diffusion the possible deformation mechanismsof high-strain-rate mechanism, k is Boltzmann's constant, and A is a superplastic materials. The tensile properties of high- constant which is principally a function of the deforma- strain-rate superplastic materials with fine grained tion mechanism.Fromthe grain size dependenceof the structures of typically 0.05 to 5,tm are characterized over superplastic strain rate from Eq. (1), also as shownin a wide range of strain rates and temperatures. Onthe Figs. I and 2, it is clearly concluded that high-strain-rate basis of the experimental results reported from the superplasticity requires a very small grain size.
Details
-
File Typepdf
-
Upload Time-
-
Content LanguagesEnglish
-
Upload UserAnonymous/Not logged-in
-
File Pages16 Page
-
File Size-