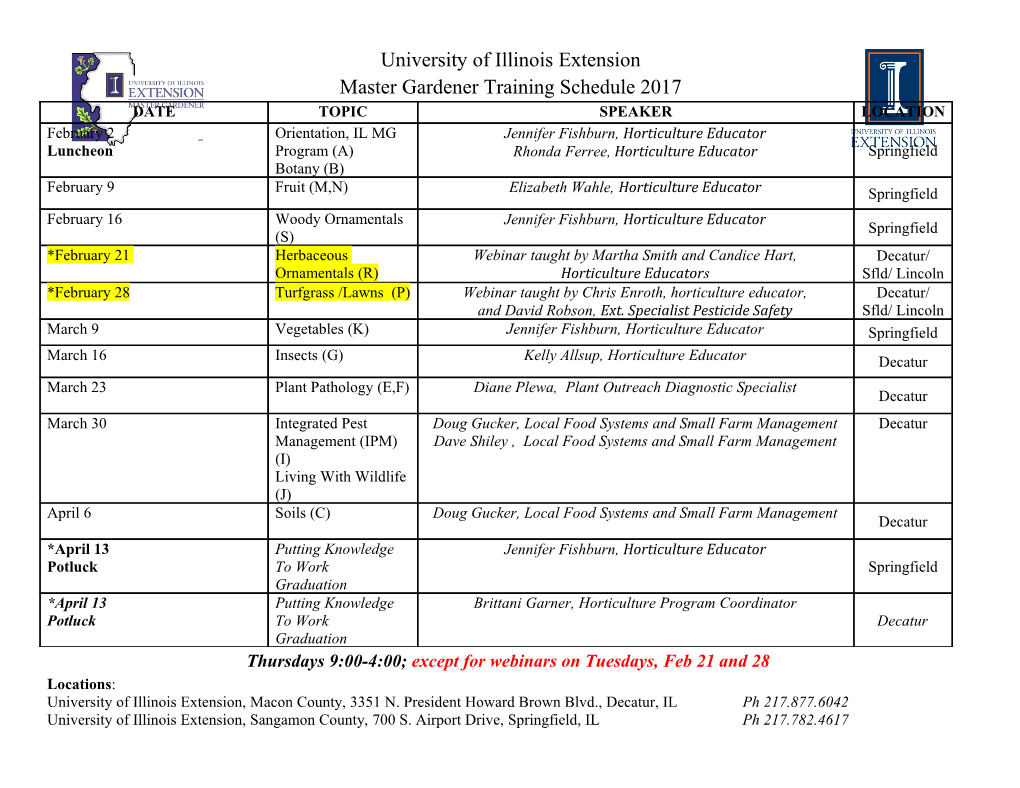
Planetary Penetrators for Sample Return Missions Chad A. Truitt A thesis submitted in partial fulfillment of the requirements for the degree of Master of Science University of Washington 2016 Committee: Robert M. Winglee Michael McCarthy Erika Harnett Program Authorized to Offer Degree: Earth and Space Sciences © Copyright 2016 Chad A. Truitt University of Washington Abstract Planetary Penetrators for Sample Return Missions Chad A. Truitt Chair of the Supervisory Committee: Professor Robert M. Winglee Earth and Space Sciences Sample return missions offer a greater science yield when compared to missions that only employ in situ experiments or remote sensing observations, since they allow the application of more complicated technological and analytical methodologies in controlled terrestrial laboratories that are both repeatable and can be independently verified. The successful return of extraterrestrial materials over the last four decades has contributed to our understanding of the solar system, but retrieval techniques have largely depended on the use of either soft-landing, or touch-and-go procedures that result in high ΔV requirements, and return yields typically limited to a few grams of surface materials that have experienced varying degrees of alteration from space weathering. Hard-landing methods using planetary penetrators offer an alternative for sample return that significantly reduce a mission’s ΔV, increase sample yields, and allow for the collection of subsurface materials, and lessons can be drawn from previous sample return missions. The following details progress in the design, development, and testing of penetrator/sampler technology capable of surviving subsonic and low supersonic impact velocities (<700 m/s) that would enable the collection of geologic materials using tether technology to return the sample to a passing spacecraft. The testing of energy absorbing material for protecting the sample, as well as the design evolution and field testing of the penetrator are discussed. It is shown through field testing that penetrators at speeds between 300-600 m/s (~Mach 1-2) can penetrate into the ground to depths of 1-2 m with overall structural integrity maintained. Four series of test flights are discussed that demonstrate the arc of the research from penetrator survivability, to successful sample collection and ejection of the sample return container. The potential for metamorphic effects during sampling is discussed along with future work that will assist in defining parameters for selecting appropriate primitive bodies for future sample return missions. TABLE OF CONTENTS List of Figures ................................................................................................................................ iii List of Tables .................................................................................................................................. v Chapter 1. Sample Return and Spacecraft ...................................................................................... 1 1.1 The Importance of Sample Return ............................... Error! Bookmark not defined. 1 1.2 Samp;le Return Methods................................................................................................. 3 1.3 Penetrators and Space Exploration ............................ Error! Bookmark not defined. 11 Chapter 2. Sample Return Systems for Extreme Enviroments (SaRSEE).................................... 15 2.1 SaRSEE Mission Concept............................................................................................. 15 2.2 Penetrator Design .......................................................................................................... 20 Chapter 3. Field Testing…………………………………………………………………………29 3.1 Black Rock, Nevada................................................... 2Error! Bookmark not defined. 9 3.2 Ione, CA: Series 1 ........................................................................................................ 33 3.3 Ione, CA: Series 2…………………………………………………………………….37 3.4 Ione, CA: Series 3…………………………………………………………………….46 Chapter 4. The Potential for Metamorphic Effects……………………………………………...50 4.1 Impact Metamorphism…………………………………………………………………50 4.2 Impact Velocity and Pressure………………………………………………………….52 4.3 Observed Impact Effects in the Sandstone…………………………………………….55 i Chapter 5. Discussion…………………………………………………………………………...61 5.1 The Orange Ring………………………………………………………………………..61 5.2 Penetration Depth and Self Ejection……………………………………………………64 5.3 Future Work…………………………………………………………………………….65 Chapter 6. Conclusion…………………………………………………………………………...67 Bibliography ................................................................................................................................. 71 ii LIST OF FIGURES Figure 1.1. Abundances of primitive material in the inner solar system [4]……………………..4 Figure 1.2. Lunar sample return yields for the Apollo and Luna programs [9]………....……….6 Figure 1.3. Artist’s concept of the Philae lander after separating from the Rosetta spacecraft [11]……………………………………………………..6 Figure 1.4. Artist’s concept of the OSIRIS-REx spacecraft deploying the TAGSAM [14][15]…………………………………………………....8 Figure 1.5. Artist’s concept of Hayabusa2 during touch-and-go sampling [18]………………....9 Figure 1.6. Artist’s concept of Genesis with its collection arrays deployed [19]……………….10 Figure 1.7. Artist’s concept of the Stardust spacecraft approaching Comet81P/ Wild 2 [20]…..10 Figure 1.8. The T-12 “Cloudmaker” and instrumented penetrators [21]……………...………...12 Figure 1.9. Schematic for the Mars-96 penetrator [22]; schematic for the DS-2 probe [24]……13 Figure 1.10. JAXA’s LUNAR-A spacecraft [21][25][26]………………………………………13 Figure 2.1. Artist’s concept of the spacecraft deploying a tethered penetrator…………………16 Figure 2.2 Artist's concept of sampling during the impact process……………………………..17 Figure 2.3 Artist’s concept showing the SRC extraction……………………………………….17 Figure 2.4. Mass ratio comparisons between fly-by and orbital sampling [30]………………...19 Figure 2.5. Artist's concept of possible spacecraft configurations……………………………...19 Figure 2.6. The Aluminum Rocket Prototype…………………………………………………..20 Figure 2.7. Simple schematic of the SaRSEE penetrator……………………………………......21 Figure 2.8. Aluminum honeycomb cells collapse in concertina-like manner…………………...22 Figure 2.9. A plot of compression testing results……………………………………………….23 Figure 2.10. A machined nose cone and crumple zone…………………………………………24 Figure 2.11. Comparison diagram showing the nose cone design for the first and second flight series…………………………………………….…25 Figure 2.12. Relative flow model created in SolidWorks……………………………………....26 Figure 2.13. The first two-stage penetrator………………………………………………….….27 Figure 2.14. The two-stage penetrator showing the out-board motors……………………….…28 Figure 3.1. The first SaRSEE penetrator…………………………………………………….….31 iii Figure 3.2. Gravedigger 1 post impact……………………………………………………….….31 Figure 3.3. The test flight of Gravedigger 2…………………………………………………….32 Figure 3.4. The nose cone from Gravedigger 2…………………………………………………32 Figure 3.5. The center-bored feed port, steel nose cone………………………………………...34 Figure 3.6. The triple feed ports, steel nose cone…………………………………………….....35 Figure 3.7. Launch of the clustered, two-stage penetrator………………………………………36 Figure 3.8. The penetrator embedded to a depth of ~0.9 meters……………………………….36 Figure 3.9. The third evolution of penetrating nose cones…………………………………...…37 Figure 3.10. A Google Earth view of the impact area……………………………………….….39 Figure 3.11. The impact site…………………………………………………………………….40 Figure 3.12. The removal of the main body of the penetrator……………………………...…..41 Figure 3.13. The recovered penetrator………………………………………………………….41 Figure 3.14. The recovered penetrator cross sectioned…………………………………………43 Figure 3.15. Crumple zone and SRC deformation……………………………………………..44 Figure 3.16. The exposed core sample……………………………………………………….…45 Figure 3.17. A portion of the core sample removed from the penetrator………………………45 Figure 3.18. The redesigned penetrator…………………………………………………...……46 Figure 3.19. The penetrator system ready for flight……………………………………………47 Figure 3.20. The impact site and ejected SRC……………………………………………...….48 Figure 3.21. Still frames from a video of the impact………………………………………...…48 Figure 3.22. The recovered SRC………………………………………………………………..49 Figure 3.23. The SRC opened revealing the stratified sample………………………………….49 Figure 4.1. Metamorphic facies diagrams…………………………………………………….…51 Figure 4.2. Impact velocity estimates for the sandstone shot…………………………………...54 Figure 4.3. Google Earth image and geologic map of the impact site……………………….….56 Figure 4.4. Thin sections from the control and sampled sandstone…………………………......58 Figure 4.5. Magnified regions of the same thin sections………………………………….….…59 Figure 5.1. The orange ring observed in the core sample…………………………………….…61 Figure 5.2. Close up of material missing from the nose cone……………………………….….63 Figure 5.3. The evolution of discoloration and ring formation………………………………....63 iv LIST OF TABLES Table 1.1. Representative compositions of select extraterrestrial bodies [2]… .......... .…..2 Table 2.1. Representative comparison of escape and orbital velocities of select minor bodies [30]………………………………….…..…15 Table 2.2. Test sample modification descriptions………………...………………...……23 v ACKNOWLEDGEMENTS Funding for this study was provided by NASA Innovative Advanced Concepts under grants NNX12AR02G and NNX13AR37G. As with all efforts in space exploration, the completion of these
Details
-
File Typepdf
-
Upload Time-
-
Content LanguagesEnglish
-
Upload UserAnonymous/Not logged-in
-
File Pages85 Page
-
File Size-