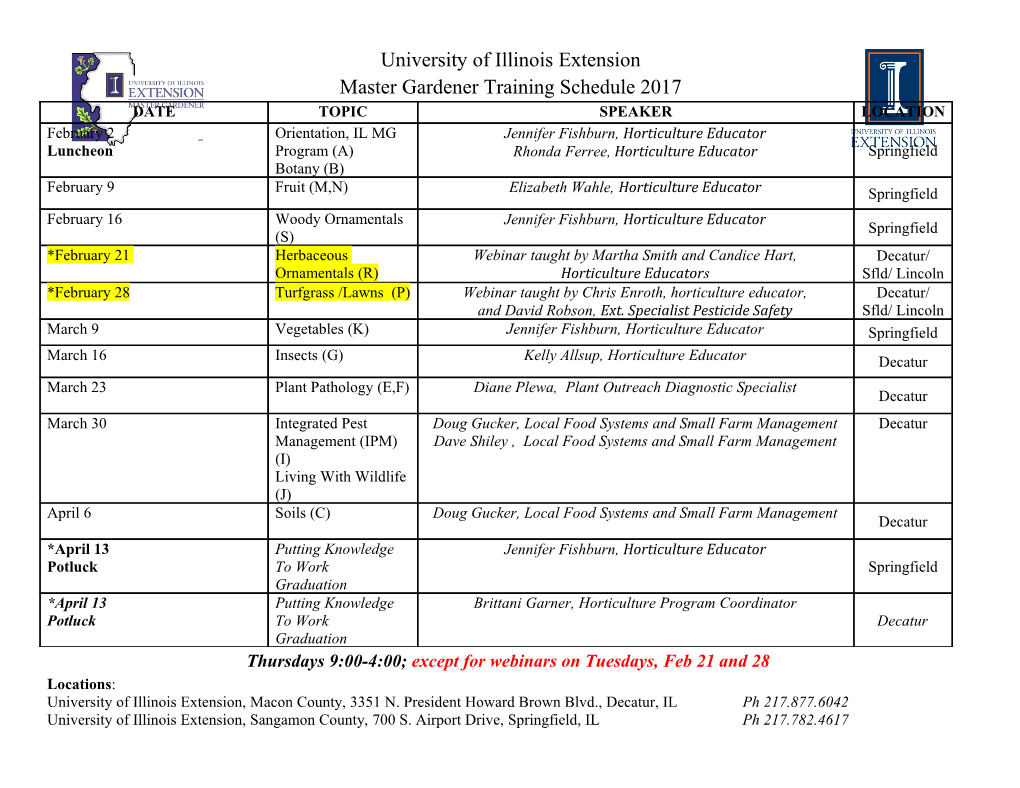
Radial Velocity Spectrograph Bean et al. The Case for an Ultra-High Precision Radial Velocity Spectrograph at Gemini Jacob L. Bean1, Andrew Szentgyorgyi1, Drake Deming2, & David Latham1 1 Harvard-Smithsonian Center for Astrophysics 2 NASA/Goddard Space Flight Center contact: [email protected] We advocate here for the implementation of a next-generation optical spectrograph capable of measuring differential radial velocities to a precision of 9 cm s−1, which is equal to the ve- locity semi-amplitude induced on the Sun by the Earth. The primary anticipated use for this instrument is the discovery and characterization of planets with masses less than 10 M⊕, including potentially habitable planets, as part of both standalone surveys, and in conjunc- tion with transit surveys and transit spectroscopy efforts. The instrument would ideally be installed at the Gemini North telescope to enable Kepler follow-up, and so as to not conflict with similar planned instruments on large telescopes in the southern hemisphere. Background Doppler spectroscopy has arguably been the most important observational technique in the short history of the field of exoplanet science, and the technique could continue to play a critical role in this field despite the advances made with other methods. One reason for this is that radial velocity surveys yield a more statistically complete sample of exoplanets than any other technique. Another reason for the ongoing importance of the radial velocity method is that radial velocity measure- ments are needed to confirm and measure the masses of planet candidates identified by transit searches. Transiting planets are the only planets for which masses and radii can be measured, and these properties together provide potent insight to the structure and bulk compositions of the planets. The fortuitous geometry of transiting planets also allows their spectra to be obtained in transmission, emission, and reflection via temporal rather than spatial sampling. In contrast, direct imaging could potentially yield the masses and spectra of planets, but will never be able to de- liver model-independent radii for planets. Therefore, the combination of transit and orbital Doppler spectroscopy provides the most complete picture of exoplanet characteristics that can be obtained short of actually sending a satellite to another system. The need for a next generation radial velocity instrument One of the main goals of the field of exoplanet science now is to ascertain the frequency, and physical and orbital characteristics of low-mass planets (defined as those with masses at least below 10 M⊕ and especially below 5 M⊕ for the purposes of this document), including those in the habitable zones of main sequence stars. The success of the combination of the radial velocity and transit techniques to study planets so far suggests that a “dynamics-based” approach could effectively address this goal over the next 10 years (Charbonneau & Deming 2007). The main aspects of this approach are: • The combination of the transit and radial velocity methods to identify and deliver masses and radii for a large, representative sample of low-mass planets so that the range of physical properties of these planets can be ascertained. Page 1 of 5 Radial Velocity Spectrograph Bean et al. • The combination of the transit and radial velocity methods to identify a few low-mass planets around bright stars for which atmospheric studies can be carried out with the techniques of transit spectroscopy. This includes the search for molecular features in the atmospheres of potentially habitable planets around M dwarfs. • The use of the radial velocity method on its own to obtain a statistically complete census of low-mass planets in the solar neighborhood so that the frequency, orbital parameters, and host star properties of low-mass planets can be investigated. The essential technology components for this approach are of course radial velocity, transit survey, and transit spectroscopy facilities that can obtain the required sensitivities. The recent launch of Kepler and its demonstrated capabilities (Borucki et al. 2010) indicates that the transit side of the statistics of low-mass planets is on firm ground. Indeed, Kepler has already identified hundreds of low-mass planet candidates in orbital periods out to a few tens of days. As the mission continues, it is likely that more transiting planet candidates will be identified at longer periods, and Kepler ultimately aims to detect a transiting Earth analog. The MEarth ongoing ground-based transit search targeting M dwarfs has already found the first transiting super- Earth around a nearby star (Charbonneau et al. 2009), and this has opened the door for the first atmospheric characterization of a low-mass planet. The proposed missions TESS1 and PLATO2 suggest that the appropriate development of transit identification of low-mass planets around bright stars is also progressing. In addition, the planned capabilities of JWST will enable atmospheric studies of transiting low-mass planets in the habitable zones around bright stars (Deming et al. 2009). Given the ongoing and expected progress on the discovery of transiting planets, the key miss- ing technology component in the dynamics-based approach to studying low-mass exoplanets are appropriate radial velocity facilities. In the last 15 years the techniques for Doppler spectroscopy have progressed to the point that precisions of 1 – 2 m s−1 are routinely obtained on bright stars (e.g., Howard et al. 2009; Bouchy et al. 2009), and precisions of 60 – 80 cm s−1 have been ob- tained in a few select cases (e.g., Mayor et al. 2009). This level of precision enables the detection of planets with masses down to approximately 5 M⊕ with periods up to a few tens of days around solar-type stars, and down to a few M⊕ in similar periods around M dwarfs. Figure 1 shows the known planets detected with the radial velocity method and the detection limits corresponding to different levels of precision. Despite the past progress on Doppler spectroscopy techniques, the radial velocity detection of low-mass planets orbiting in the habitable zones of solar-type stars requires an approximately order of magnitude improvement over existing capabilities. In addition, the most interesting planets already identified with Kepler, to say nothing of those planets still to be identified during the nominal mission, can not be confirmed and weighed with existing facilities because the radial velocity sig- nals are too small and/or the host stars are too faint (note that Kepler looks at a field from +37.5◦ to +52.5◦ in declination). TESS and PLATO will have the same problem if they fly. Furthermore, the recent super-Earth planet discovered by the MEarth facility was only possible to be confirmed and have its mass measured because it was observable by southern hemisphere facilities. Exclusively northern hemisphere candidates identified by MEarth in the future will be extremely challenging to perform the necessary follow-up radial velocity measurements for, primarily because of the faint- ness of their host stars. Ultimately, the lack of follow-up capabilities for ongoing and future transit 1see http://space.mit.edu/TESS 2see http://www.lesia.obspm.fr/perso/claude-catala/plato web.html Page 2 of 5 Radial Velocity Spectrograph Bean et al. Figure 1: Comparison of planet masses and orbital periods with radial velocity detection limits. The filled circles are the known exoplanets detected with the radial velocity method. The crosses are the eight Solar System planets. The lines give the planet mass corresponding to a velocity −1 −1 semi-amplitude of 1 m s (dashed lines) and 9 cm s (solid lines) for a 1.0 M⊙ star (blue lines) and a 0.2 M⊙ star (orange lines). searches suggests that the future atmospheric characterization of low-mass transiting planets with JWST hinges critically on the development of new radial velocity capabilities, especially in the northern hemisphere Instrument requirements Clearly, a next-generation specialized radial velocity instrument is needed. We advocate for a such an instrument with an achievable precision given by the velocity semi-amplitude induced on the Sun by the Earth: 9 cm s−1. This is the minimum precision needed to detect a true Earth analog as part of a survey (i.e., not an “all eggs in one basket” intense campaign on one or two stars), and would also at least technically enable detection of analogs of all the other Solar System planets except Mercury and Mars. In addition, this level of precision would enable confirmation and mass measurement for many candidate transiting low-mass planets identified by Kepler and other existing or planned surveys to be accomplished as part of routine operations. We acknowledge the possibility that ultimately the radial velocity technique may be limited by astrophysical noise sources like pulsations, granulation, and magnetic field effects, and that 9 cm s−1 may be difficult to impossible to obtain in practice. However, stellar variability at this level is poorly understood, and current instruments have been used up to their technical limits (sig- nificantly less than 1 m s−1 for HARPS) for quiet stars without reaching the astrophysical noise floor. Furthermore, many people in the community feel that long-term precisions of at least about Page 3 of 5 Radial Velocity Spectrograph Bean et al. 30 cm s−1 can be obtained for solar-type stars with an appropriate observing strategy3. In light of this, we propose that a next generation radial velocity instrument should be designed so that the technical issues are not the limiting factor in approaching the detection of an Earth analog. An 8 m class telescope is required for an instrument designed to obtain a new level of sensitivity because obtaining just a photon-limited precision of 9 cm s−1 requires a huge number of counts to be recorded.
Details
-
File Typepdf
-
Upload Time-
-
Content LanguagesEnglish
-
Upload UserAnonymous/Not logged-in
-
File Pages5 Page
-
File Size-