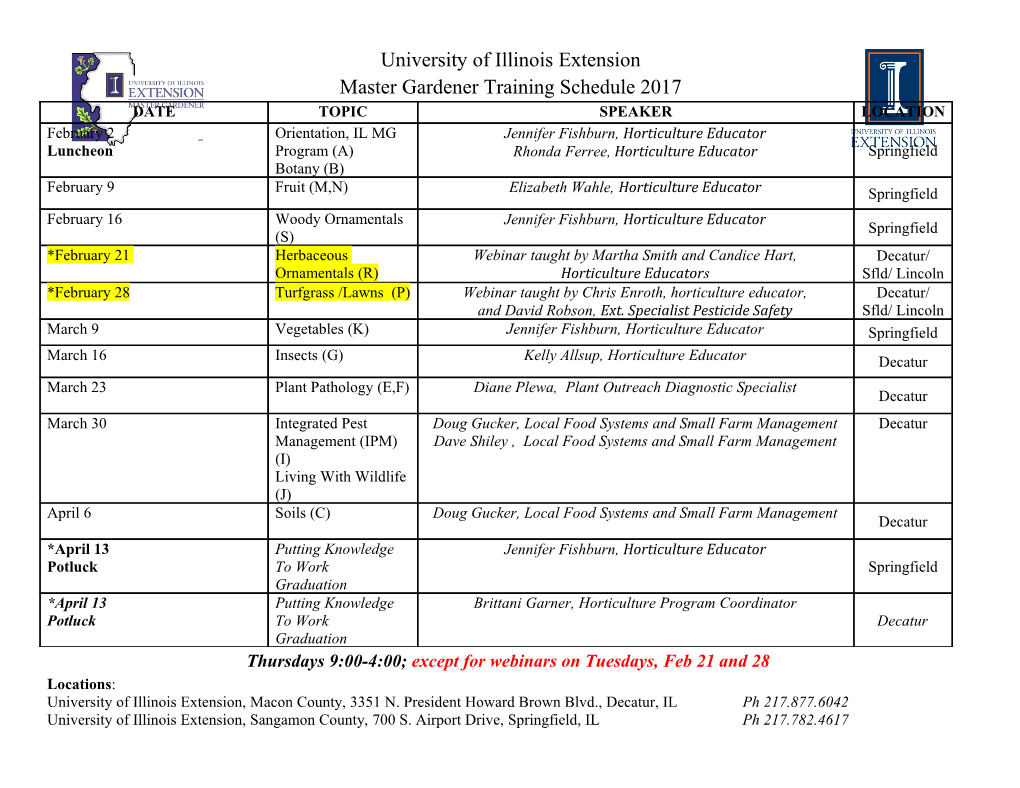
FAU Institutional Repository http://purl.fcla.edu/fau/fauir This paper was submitted by the faculty of FAU’s Harbor Branch Oceanographic Institute. Notice: ©1997 Inter-Research Science Center. This manuscript is available at http://www.int-res.com/ and may be cited as: Maldonado, M., George, S. B., Young, C. M., & Vaquerizo, I. (1997). Depth regulation in parenchymella larvae of a demosponge: relative roles of skeletogenesis, biochemical changes and behavior. Marine Ecology Progress Series, 148, 115-124. doi:10.3354/meps148115 MARINE ECOLOGY PROGRESS SERIES Vol. 148: 115-124, 1997 Published March 20 Mar Ecol Prog Ser /"'> Depth regulation in parenchymella larvae of a demosponge: relative roles of skeletogenesis, biochemical changes and behavior Manuel Maldonado", Sophie B. George··, Craig M. Young, Isabel Vaquerizo Department of Larval Ecology, Harbor Branch Oceanographic Institution, 5600 U.S. Hwy 1 North, Fort Pierce, Florida 34946, USA ABSTRACT: To assess factors that influence depth regulation of sponge larvae, we documented onto­ genetic changes in larval size and shape, lipid and protein content, skeletal development, and photo­ response in Sigmadocia caerulea, a shallow-water demosponge in the order Haplosderida. We also measured size and biochemical differences among larvae from different parents to determine how depth regulation might vary across the population. Larvae were photonegative during the entire free­ swimming period. Younger larvae swam faster than older larvae, but older larvae swam away from light for greater time and distances. Sinking rates of anesthetized larvae increased as a function of age, not because of lipid depletion or shape changes, but because addition of spicules increased density. Neither lipid nor protein changed significantly during larval life, but protein content increased abruptly just after settlement. Minor differences in length and protein content among offspring from different parents had no apparent effect on depth regulation. Both active movement and passive sinking play roles in moving late-stage larvae towards the sea floor, but increase in larval spicular mass appears to be the most important factor. KEY WORDS: Depth regulation' Larval behavior· Larval composition' Photobehavior· Sponge larvae INTRODUCTION vae experience an increase in mass and a commensu­ rate reduction in buoyancy near the end of larval life. Marine invertebrate larvae must generally have The relative roles of active behaviors and passive sink­ mechanisms for remaining suspended while dispers­ ing have been discussed for crustacean (Sulkin 1984) ing and for returning to the bottom prior to settlement. and echinoderm (Emlet 1983) larvae, but the proxi­ Thorson (1964) proposed that ontogenetic changes in mate factors influencing sinking rate have seldom behavior, particularly photoresponses, often mediate been analyzed. the transition from the plankton to the benthos. How­ Passive sinking rate of a small larva heavier than ever, changes in the buoyancy of larvae can also playa seawater is determined by the balance between the significant role. For example, ophiuroid larvae often upward force of drag and the downward force of grav­ undergo metamorphosis in the water column, there­ ity pulling on the larva's mass. If we assume a Reynolds after falling to the bottom passively as heavy ossicles number (Re) less than 1. a spherical larva of radius a develop and ciliary swimming ability is lost (Emlet sinks at a terminal velocity U calculated as 1983). Anecdotal observations indicate that many lar- where II is the dynamic viscosity of seawater, P is the Present addresses: density of the larva, Pois the density of seawater and g 'Department of Aquatic Ecology, Centro de Estudios Avan­ is the gravitational constant (Vogel 1981). Drag can be zados de Blanes (eSIC), Camino de Santa Barbara sin, E­ increased or decreased during ontogeny by shape 17300 Blanes, Girona, Spain. E-mail: [email protected] ..Department of Biology, Georgia Southern University, changes, though such differences tend to be small at Statesboro, Georgia 30460-8042, USA low Reynolds numbers. For example, at Re = 1, a pro- © Inter-Research 1997 Resale of full article not permitted 116 Mar Ecol Prog Ser 148: 115-124, 1997 late spheroid twice as long as its diameter has a in the Indian River Lagoon near Fort Pierce, Florida, slightly lower drag coefficient (11.17) than a sphere USA, during August and September 1994 and 1995. (11.69) of the same volume (Happel & Brenner 1973, Adult sponges in this region are light blue, usually Vogel 1981). Larvae may undergo ontogenetic erect and branched, and up to 30 cm tall. changes in density by metabolizing buoyant lipids, Larval release often occurred spontaneously shortly synthesizing heavier proteins, or secreting skeletal after collection of ripe adults. Larval release was also elements. triggered artificially by exposing adults to air for a few Sponges have no integrated nervous system in either seconds (Maldonado & Young 1996). Larvae were cul­ the adult or larval stage, so their behaviors should be tured at room temperature in glass or polystyrene very limited. Nevertheless, it has been reported that bowls of 0.45 urn filtered seawater that was replaced sponge larvae switch the signs of their phototactic and daily (salinity = 34 %0, temperature = 22 to 24°C). geotactic responses with age (e.g. Wilson 1935, Hart­ Larval size and shape. All experimental data were man 1958, Warburton 1966, Berqquist et al. 1970, 1979, checked for normality (Kolmogorov-Smirnov test) and Fell 1974, Wapstra & van Soest 1987). Forward (1988) homoscedasticity (Levene median test) prior to analy­ has discussed potential laboratory artifacts associated sis. When, after appropriate transformation, data failed with measurements of phototaxis, and Sulkin (1990) either normality or homoscedasticity tests, they were has encompassed the conditions under which such analyzed by appropriate non-parametric methods. measurements are useful. Although the responses to Changes in larval length and larval shape over time light and gravity in the laboratory may not resemble were determined by diqitizinq the images of live lar­ the actual behaviors exhibited in the field, changes in vae with a Microcomp image analysis system linked to response between stages are nevertheless indicative of a Zeiss dissecting microscope. physiological processes taking place within the larvae. Lengths of 413 larvae were used to estimate the lar­ All known sponge larvae are lecithotrophic and pre­ val size distribution at the population level. Differences sumably metabolize storage products durinq the in length among 5 age groups of larvae (2, 6, 12, 24, course of larval life, so it is also possible that density and 30 h old larvae; N =50,25,50,87, and 76, respec­ changes help larvae move toward the bottom. There tively) from a single parent were tested by l-way are, however, no data Whatsoever on the biochemical analysis of variance. Differences in the lengths of 6 h composition of larval or juvenile demosponges. old larvae obtained from 5 different parents collected In this study, we document various changes that from the same population were also examined by 1­ occur during larval life of Sigmadocia caerulea (Hech­ way analysis of variance (N = 25). tel 1965), a shallow-water demonsponge in the order Overall changes in larval shape over time were Haplosclerida. This species is common throughout the assessed by use of the circularity index (C) of Turon & Caribbean and on the Pacific coast of Panama (Zea Becerro (1992): C = A.IAp , where A. is the area of the 1987). Our goal was to measure ontogenetic changes object, and A p is the area of a circle with a perimeter in the various parameters that could influence the abil­ equivalent to that of the object. Areas and perimeters ity of larvae to find the bottom at the end of larval life, projected by larvae on the bottoms of the containers namely: (1) changes in larval shape and size that might were used to calculate the larval circularity index. Dif­ influence drag, (2) changes in photoresponse and ferences in the circularity index among 5 age groups swimming speed that might cause larvae to move (2,6, 12,24, and 30 h old larvae; N = 50,27,50,51, and away from the surface, and (3) changes in biochemical 26, respectively) were tested by l-way analysis of vari­ composition [i.e. relative amounts of proteins and ance. buoyant lipids) and in secreted inorganic spicules that Larval swimming, orientation, and sinking. The could influence larval density. In this way, we hoped to length of the swimming period under laboratory condi­ determine which factors were most important in bring­ tions was determined by monitoring larvae held in 15 ing larvae to the bottom for settlement. We also mea­ small polystyrene petri dishes over a period of 17.5 d. sured some of these parameters in larvae from differ­ Each dish contained 10 larvae in 30 ml of 0.45 urn fil­ ent parents to determine how the factors influencing tered seawater (salinity = 34 %0, temperature = 22 to depth regulation might vary across a population. 24°C). Phototactic and photokinetic responses were investi­ gated by recording movements of 6 and 30 h old larvae MATERIALS AND METHODS (N = 25 at each age) in the presence of a bright unidi­ rectional light. As we were only interested in the Collection and maintenance of adults and larvae. intrinsic ability of larvae to respond to light, we made We collected ripe individuals of Sigmadocia caerulea no attempt to mimic the angular light distributions in from boulders and cobbles between 0.5 and 2 m deep the field (Forward 1988). Instead, we used a standard- Maldonado et al.: Depth regulation in demosponge parenchymella larvae 117 ized light source for all tests. The light source was a 3.4 % ammonium formate, mounted on tared alu­ 150 W, cold fiber-optic incandescent light filtered minum boats and dried to constant weight at 80°C. through a neutral density diffuser.
Details
-
File Typepdf
-
Upload Time-
-
Content LanguagesEnglish
-
Upload UserAnonymous/Not logged-in
-
File Pages11 Page
-
File Size-