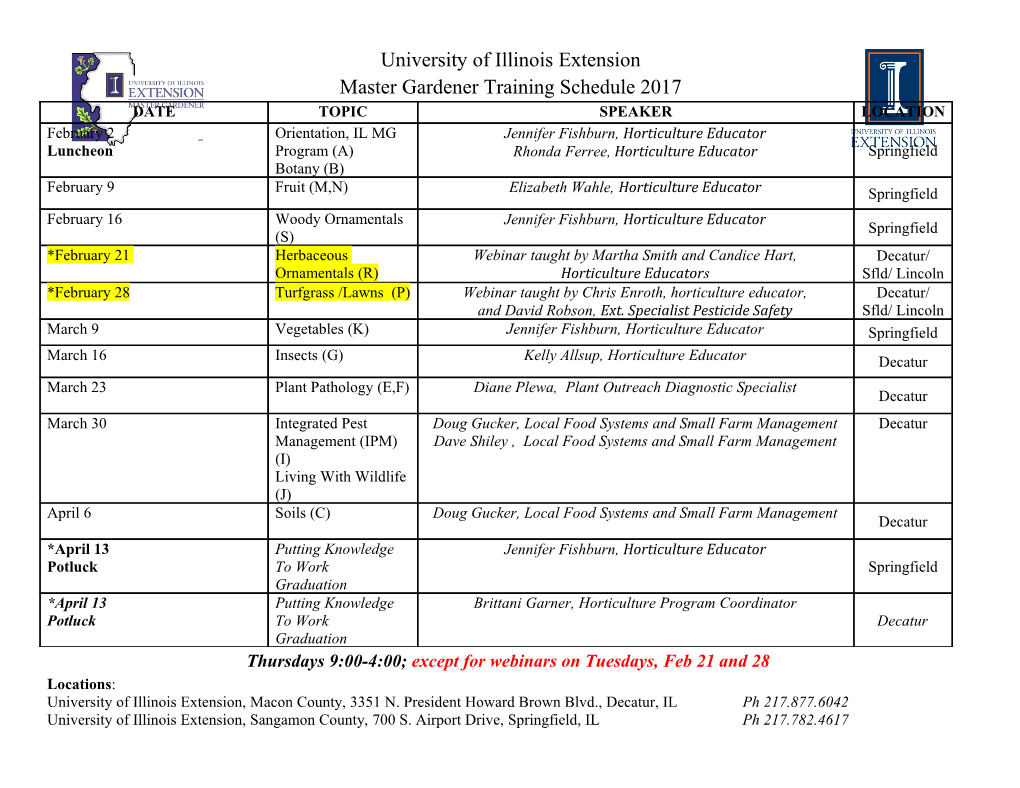
Journal of Vegetation Science 13: 301-314, 2002 © IAVS; Opulus Press Uppsala. Printed in Sweden - Environmental variation, vegetation distribution, carbon dynamics, and water/energy exchange - 301 Environmental variation, vegetation distribution, carbon dynamics and water/energy exchange at high latitudes McGuire, A.D.1*; Wirth, C.2; Apps, M.3; Beringer, J.4; Clein, J.5; Epstein, H.6; Kicklighter, D.W.7; Bhatti, J.3; Chapin III, F.S.5; de Groot, B.3; Efremov, D.8; Eugster, W.9; Fukuda, M.10; Gower, T.11; Hinzman, L.12; Huntley, B.13; Jia, G.J.6; Kasischke, E.14; Melillo, J.7; Romanovsky, V.15; Shvidenko, A.16; Vaganov, E.17 & Walker, D.5 1U.S. Geological Survey, Alaska Cooperative Fish and Wildlife Research Unit, University of Alaska Fairbanks, Fairbanks, AK 99775, USA; 2Max-Plank Institut für Biogeochemie, Postfach 100164, D-07701 Jena, Germany; 3Canadian Forest Service, Northern Forestry Center, Edmonton, Alb., T6H 3S5, Canada; 4School of Geography and Environmental Science, Monash University, Clayton, Vic 3800, Australia; 5Institute of Arctic Biology, University of Alaska Fairbanks, Fairbanks, AK, 99775, USA; 6Dept. of Environmental Sciences, University of Virginia, Charlottesville, VA 22904, USA; 7The Ecosystems Center, Marine Biological Laboratory, 7 MBL Street, Woods Hole, MA 02543, USA; 8Far Eastern Forestry Research Institute, Khaborovsk, 680030, Russia; 9University of Bern, Geographical Institute, Hallerstrasse 12, 3012 Bern, Switzerland; 10Institute of Low Temperature, Hokkaido University, N 19 W8 Sapporo, 060 Japan; 11Dept. of Forest Ecology and Management, Univer- sity of Wisconsin, Madison, WI 53706, USA; 12Institute of Northern Engineering, University of Alaska Fairbanks, Fairbanks, AK 99775, USA; 13Environmental Research Centre, University of Durham, Durham DH1 3LE, United Kingdom; 14Dept. of Geography, University of Maryland, College Park, MD 20742, USA; 15Geophysical Institute, University of Alaska Fairbanks, Fairbanks, AK 99775, USA; 16International Institute for Applied Systems Analysis, A-2361 Laxenburg, Austria; 17Institute of Forestry, Russian Academy of Sciences, Krasnoyarsk, 660036, Russia; *Corresponding author; E-mail [email protected] Abstract. The responses of high latitude ecosystems to global Introduction change involve complex interactions among environmental variables, vegetation distribution, carbon dynamics, and water As high latitude ecosystems contain ca. 40% of the and energy exchange. These responses may have important world’s soil carbon inventory that is potentially reactive consequences for the earth system. In this study, we evaluated in response to near-term climate change (McGuire et al. how vegetation distribution, carbon stocks and turnover, and water and energy exchange are related to environmental 1995), functional and structural changes in high latitude variation spanned by the network of the IGBP high latitude ecosystems have the potential to influence carbon transects. While the most notable feature of the high latitude exchange with the atmosphere (Smith & Shugart 1993; transects is that they generally span temperature gradients McGuire & Hobbie 1997; McGuire et al. 2000). Regions from southern to northern latitudes, there are substantial affected by permafrost are especially vulnerable to differences in temperature among the transects. Also, along climate change because of altered drainage. Thermakarst each transect temperature co-varies with precipitation and lakes and wetlands may become large sources of methane photosynthetically active radiation, which are also variable (Reeburgh & Whalen 1992; Zimov et al. 1997). among the transects. Both climate and disturbance interact to Reductions in the water table of tundra ecosystems influence latitudinal patterns of vegetation and soil carbon storage among the transects, and vegetation distribution appears substantially enhance the release of carbon from high to interact with climate to determine exchanges of heat and latitude soils (Christensen et al. 1998). In contrast, earlier, moisture in high latitudes. Despite limitations imposed by the longer and warmer growing seasons may increase data we assembled, the analyses in this study have taken an production in tundra and boreal forest to increase carbon important step toward clarifying the complexity of interactions sequestration (Chapin et al. 1995; Frolking et al. 1996; among environmental variables, vegetation distribution, carbon Oechel et al. 2000; McGuire et al. 2000). The replacement stocks and turnover, and water and energy exchange in high of tundra with boreal forest might initially decrease but latitude regions. This study reveals the need to conduct eventually increase carbon storage in high latitudes coordinated global change studies in high latitudes to further (Smith & Shugart 1993). Disturbance in the boreal elucidate how interactions among climate, disturbance, and vegetation distribution influence carbon dynamics and water forest region may substantially influence regional carbon and energy exchange in high latitudes. exchange with the atmosphere (Kasischke et al. 1995; Kurz & Apps 1999; Schulze et al. 1999; Shvidenko & Keywords: Boreal; Climate; Disturbance; Energy; Gradient; Nilsson 2000; Wirth et al. in press). The functional and Tundra. structural responses of carbon storage in high latitude 302 McGuire, A.D. et al. ecosystems have important implications for the rate of Together, these transects provide a network for improving CO2 accumulation in the atmosphere and international our understanding of controls over vegetation dynamics, efforts to stabilize the atmospheric concentration of carbon dynamics, and water and energy exchange in CO2 (Smith & Shugart 1993; McGuire & Hobbie 1997; high latitudes. In this paper, we take a step toward McGuire et al. 2000). clarifying the complexity of these interactions by Responses of high latitude ecosystems to global evaluating how vegetation distribution, carbon stocks change have the potential to influence water and energy and turnover, and water and energy exchange are related exchange with the atmosphere in several ways. Sharp to environmental variation spanned by the network of discontinuities in ecosystem structure, such as the forest- the IGBP high latitude transects. We first briefly describe tundra boundary, are hypothesized to strongly influence the high latitude transects in the network and describe temperature (Eugster et al. 2000). In comparison to the sources of data used in our analyses. We then examine boreal forest, snow-covered tundra and boreal wetlands the environmental variation spanned by the transects have a much higher albedo, absorb less radiation, and and document how the environmental variation relates warm the atmosphere less than boreal forest (Betts & to vegetation distributed along each of the transects. Ball 1997; Chapin et al. 2000). Spatial variation in Next, we compare patterns of carbon storage, net primary vegetation within arctic tundra may have climatic effects production (NPP), and carbon turnover among the that extend beyond arctic tundra (e.g., see Lynch et al. transects in relation to environmental variation, 1999). The open woodlands of boreal Eurasia that result vegetation distribution, and disturbance. We then from repeated surface fires may have a ratio of sensible evaluate how water and energy exchange relates to to latent heat that is a factor of 8 higher than closed environmental variation and vegetation distribution. stands (Schulze et al. 1999; Rebmann et al. in press). During the growing season, deciduous stands have twice the albedo, i.e., reflect twice the short-wave radiation, General description of the High Latitude Transects and have 50% to 80% higher evapotranspiration than coniferous forests (Baldocchi et al. 2000). An expansion The Far East Siberia Transect of boreal forest into regions now occupied by tundra has the potential to reduce albedo and increase spring energy The Far East Siberia Transect (FEST) (also known absorption to enhance atmospheric warming (Chapin et as the ‘Northeast Eurasian transect’ and the ‘Yakutsk al. 2000). Other effects that may enhance atmospheric transect’) is a north-south transect centred on ca. 135∞ E warming include earlier snow melt, which is likely to that has been designed with respect to temperature decrease springtime albedo, and expansion of shrub variability between 52∞ and 70∞ N (Fig. 1). We have tundra, which in summer is likely to decrease evaporation identified 36 study sites in this transect between 120∞ losses because of lower evaporation from mosses (Chapin and 145∞ E where numerous studies have estimated et al. 2000) and in winter is likely to accumulate more carbon stocks of vegetation and the upper soil layers, to drifting snow during the winter and delay snow melt in understand energy, water, and carbon dynamics and to spring (Liston et al. 2002; Sturm et al. 2001). In contrast, reconstruct palaeo-environmental conditions (Hollinger responses of the disturbance regime that increase the et al. 1995, 1998; Schulze et al. 1995; Kobak et al. 1996; proportion of non-forested lands and deciduous forests Vygodskaya et al. 1997). In addition, atmospheric carbon have the potential to reduce spring energy absorption dioxide and methane concentrations have been monitored and work against atmospheric warming (Chapin et al. within this transect by aircraft sampling (Izumi et al. 2000; Eugster et al. 2000). 1993; Machida et al. 1995). Vegetation within this It is clear that the responses of high latitude eco- transect
Details
-
File Typepdf
-
Upload Time-
-
Content LanguagesEnglish
-
Upload UserAnonymous/Not logged-in
-
File Pages15 Page
-
File Size-