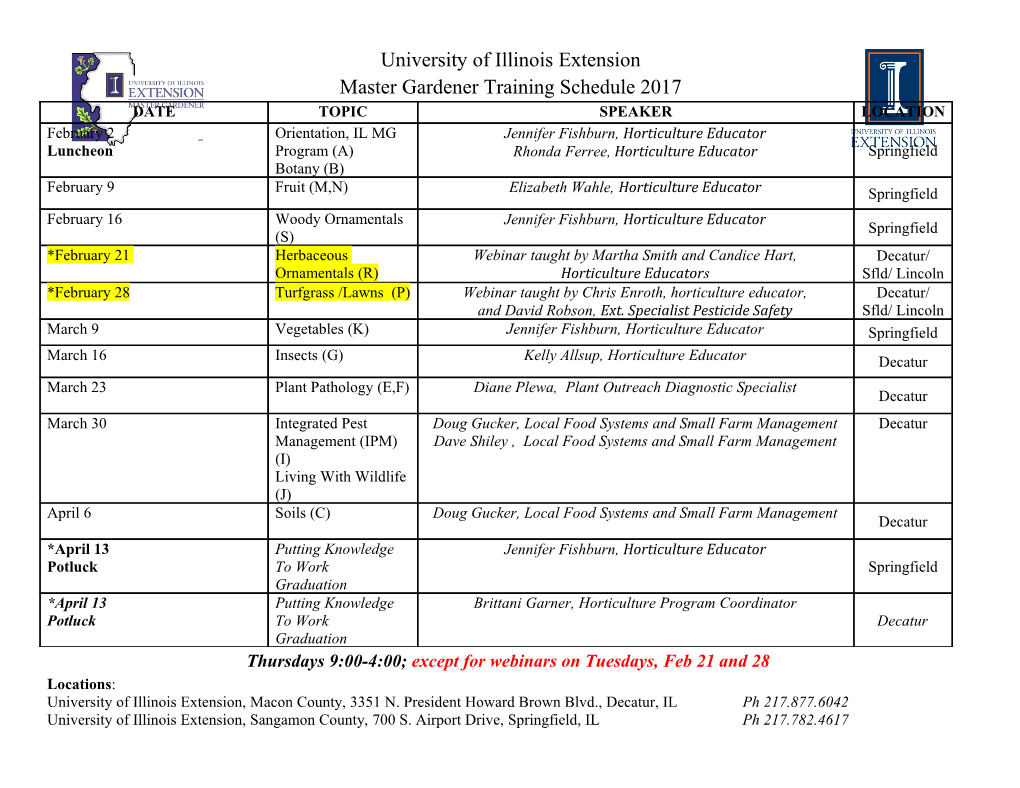
FERROUS FRICTION STIR WELD PHYSICAL SIMULATION A Dissertation Presented in Partial Fulfillment of the Requirements for the Degree Doctor of Philosophy in the Graduate School of The Ohio State University By Seth Jason Norton, M.S. ******** The Ohio State University 2006 Dissertation Committee: Approved by Dr. John Lippold, Adviser Dr. David Dickinson Adviser Dr. Charles Albright Welding Engineering Graduate Program ii ABSTRACT Traditional fusion welding processes have several drawbacks associated with the melting and solidification of metal. Weld defects associated with the solidification of molten metal may act as initiation sites for cracks. Segregation of alloying elements during solidification may cause local changes in resistance to corrosion. The high amount of heat required to produce the molten metal in the weld can produce distortion from the intended position on cooling. The heat from the electric arc commonly used to melt metal in fusion welds may also produce metal fumes which are a potential health hazard. Friction stir welding is one application which has the potential to make full thickness welds in a single pass, while eliminating fume, reducing distortion, and eliminating solidification defects. Currently the friction stir welding process is used in the aerospace industry on aluminum alloys. Interest in the process by industries which rely on iron and its alloys for structural material is increasing. While friction stir welding has been shown to be feasible with iron alloys, the understanding of friction stir welding process effects on these materials is in its infancy. This project was aimed to better that understanding by developing a procedure for physical simulation of friction stir welding. Friction stir weld material tracer experiments utilizing stainless steel markers were conducted with plates of ingot iron and HSLA-65. Markers of 0.0625” diameter 308 stainless steel worked well for tracing the end position of material moved by the friction stir welding tool. The markers did not produce measurable increases in the loading of the tool in the direction of travel. Markers composed of 0.25” diameter 304 stainless steel did not perform as well as the smaller markers and produced increased loads on the friction stir welding tool. The smaller markers showed that material is moved in a curved path ii around the tool and deposited behind the tool. Material near the surface is moved a greater distance as it is acted upon by the tool shoulder. A friction stir weld was made on a plate of HSLA-65 which had 0.0625” Inconel sheathed thermocouples embedded in the tool path at seven positions. Thermocouples on the top of the plate acquired data at the desired position until encountering the shoulder, at which point they were sheared by the shoulder and stirred behind the tool. Thermocouples on the bottom of the plate were deformed a relatively small amount and acquired data throughout the welding process. Heating rates calculated from the slope of the acquired temperature data show that the peak heating rate (~1100ºC on top and ~500ºC on the bottom) occurs on both the top and bottom of the weld at temperatures between 350ºC and 500ºC. An increase in the heating rate occurring at elevated temperature was associated with the transformation from ferrite to austenite. Comparison of phase transformation data acquired in rapid heating in the Gleeble® suggests that austenite transforms back to ferrite at higher temperatures in the presence of strain than in its absence. Peak temperatures on the top of the plate exceeded 1200ºC and peak temperatures acquired on the bottom exceeded 1000ºC. The heating rate method of data analysis was sensitive enough to pick up variations in the heating rate which occurred at the same frequency as the rotation rate of the tool. Hot torsion tests were conducted on both ingot iron and HSLA-65 samples. An annular sample geometry with internal and external gas quench achieved cooling rates in the hot torsion samples similar to those observed in friction stir welding. Designed experiments with varying test temperatures, number of rotations, and rate of rotation were conducted in both study alloys. A scribed line on the sample surface provided a means of measuring the distribution of strain in the sample gage sections and thermocouples on the non-rotating side of the sample allowed an estimate of the thermal profile at the application of torsion. The combination of these two features provides plots of strain versus temperature at the initiation of torsion. Localization of strain in the intercritical temperature region, measured by this means, was determined to be caused by differences in the activation energy for deformation for ferrite and austenite. Adiabatic heating due to shear strain was shown to be related to the Zener-Holloman parameter. Microstructures iii created in both the ingot iron and HSLA-65 were very similar to those observed in friction stir welds made in the same material. A method to study the effects of rapid heating and cooling along with the application of shear strain at a high rate has been developed for the simulation of friction stir weld structures. The combination of thermal data acquired from friction stir welds and hot torsion testing of the same materials has highlighted the importance of the allotropic phase transformation on material flow and local heating rate. Based on these observations nomenclature which is believed to be more representative of the regions in ferrous friction stir welds has been proposed. The fine grained zone, the intercritical zone, and the recovered zone are suggested as replacements for the stir zone, thermomechanically affected zone, and heat affected zone respectively. The hot torsion test with annular samples and gas quench offers the possibility of estimating the rate of strain at a given temperature. The ability to measure adiabatic heating due to the application of shear strain at high rates will provide data for modeling the generation of heat friction stir welds. With continued work this test will provide valuable information for mathematical modeling of the friction stir welding process. The new method is unfortunately limited by the optical pyrometer which measures temperature at the center of the sample. The range over which the pyrometer accurately reads temperature is higher than the melting temperature for aluminum, which prevents the use of Gleeble® torsion testing with the material most frequently associated with friction stir welding. iv DEDICATION Dedicated to my wife Shiloh and my 4 children: Their love and support make me strive to be a better person. v ACKNOWLEDGMENTS I would like to thank my advisor, John Lippold, for his support, guidance, and encouragement. Should I ever find myself employed in academia, advising students, I hope be able to emulate his style and ethic. I feel truly blessed to have had him as my advisor for the six years I have been at The Ohio State University. I would also like to extend thanks to Dr. Boian Alexandrov, who acted as a sounding board for me, helped with data collection and collaborated on some Gleeble testing. The Edison Welding Institute (Tim Li in particular) is acknowledged for the use of their friction stir welding machine and tools. Thanks to Dr. Bahman Zoofan for sharing his expertise and skill in radiography. The X-ray images of the friction stir welds I gave him are incredible. Dr. Suresh Babu I thank for sharing his seemingly inexhaustible knowledge of Thermocalc and for his inquisitive nature. He seemed to raise more questions than he answered. Dr. Julie Christodoulou from the Office of Naval Research provided the funding for this project, without which this work would never have been completed. I would like to thank Dr. David Dickinson, and Dr. Charlie Albright for serving on my advisory committee and reviewing this manuscript. Valuable data and materials were provided by the Naval Surface Warfare Center Carderock Division. Thanks to Jennifer Nguyen, David Forrest, Maria Posada, and Carrie Davis for providing the HSLA-65 plate and data from their experiments. Dr. Blair London, a professor at the California Polytechnic State University, is acknowledged for his suggestion to use metal sheathed thermocouple wires and his leads in finding a supplier. vi Cameron Begg, of the Campus Electron Optics Facility, deserves recognition for his help and training in acquiring electron backscatter diffraction patterns in scanning electron microscopes. Thanks to Andy Doorman and Dave Ferguson, of DSI, for answering my many questions on the use of the Hot Torsion MCU and for providing the engineering sketches of the various parts I needed to alter. I am grateful to the entire Materials Joining Metallurgy Group for their help, support, and comments; in particular Melissa Rubal, Joe Walko, and Kenny Izor, the undergraduate students who helped with Thermo-Calc and metallographic sample preparation. I would like to thank Allison Polasik, a graduate student in the Materials Science Engineering department, who volunteered to proof-read portions of my manuscript. I thank Fikry Botros and Jim Ibarra at BP for hiring me and for their patience in allowing me to finish this document before beginning my post-doctoral career. My family deserves recognition for their patience and sacrifice while I worked on this dissertation. It is great to come home to their smiling faces, hugs, and kisses. I love you all and look forward to spending more time with you once this is all over. Finally, I believe none of this would be possible without the inspiration and abilities given me by my creator. I thank God for my inquisitive nature, and the friends and family I have been blessed with. vii VITA November 29, 1974………………………… Born – Sanger, California, U.S.A. 1998…………………………………………B.S. Engineering, LeTourneau University 1998 – 1999....................................................Researcher/Lab Instructor, LeTourneau University Welding Engineering Dept.
Details
-
File Typepdf
-
Upload Time-
-
Content LanguagesEnglish
-
Upload UserAnonymous/Not logged-in
-
File Pages260 Page
-
File Size-