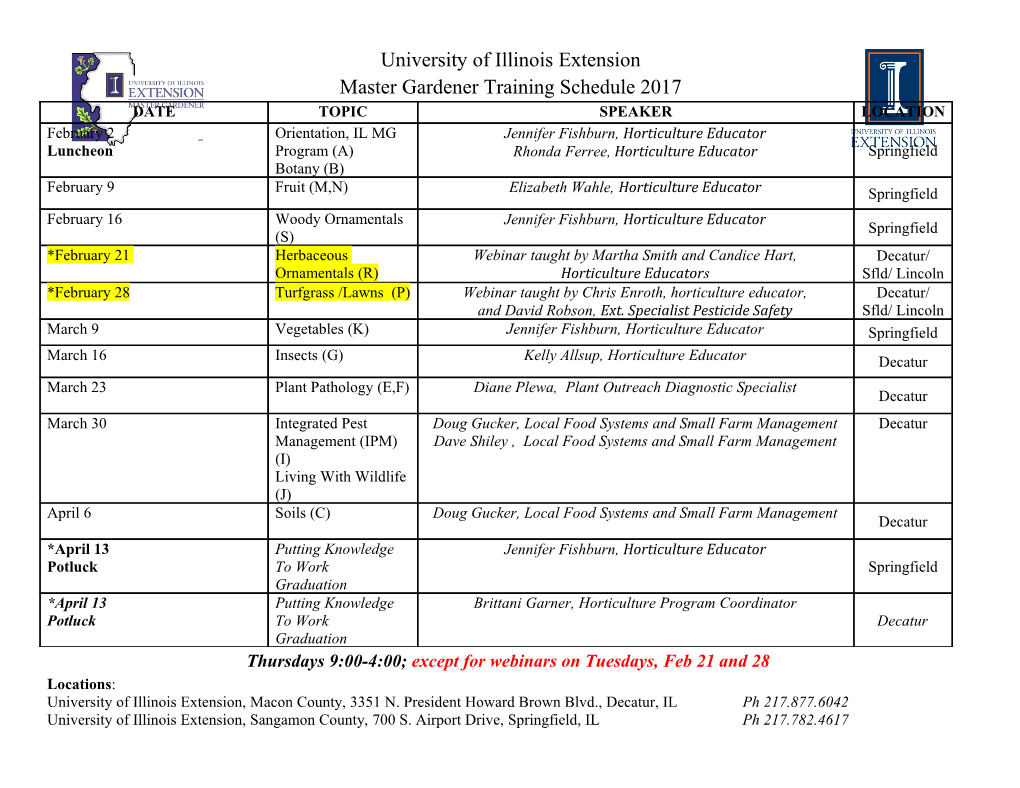
This is the author’s manuscript for publication. The publisher-formatted version may be available through the publisher’s web site or your institution’s library. Short-term oral atrazine exposure alters the plasma metabolome of male C57BL/6 mice and disrupts α-linolenate, tryptophan, tyrosine and other major metabolic pathways Zhoumeng Lin, James R. Roede, Chunla He, Dean P. Jones, Nikolay M. Filipov How to cite this manuscript If you make reference to this version of the manuscript, use the following information: Lin, Z., Roede, J. R., He, C., Jones, D. P., & Filipov, N. M. (2014). Short-term oral atrazine exposure alters the plasma metabolome of male C57BL/6 mice and disrupts α - linolenate, tryptophan, tyrosine and other major metabolic pathways. Retrieved from http://krex.ksu.edu Published Version Information Citation: Lin, Z., Roede, J. R., He, C., Jones, D. P., & Filipov, N. M. (2014). Short-term oral atrazine exposure alters the plasma metabolome of male C57BL/6 mice and disrupts α -linolenate, tryptophan, tyrosine and other major metabolic pathways. Toxicology, 326, 130-141. Copyright: © 2014 Elsevier Ireland Ltd. Digital Object Identifier (DOI): doi:10.1016/j.tox.2014.11.001 Publisher’s Link: http://www.sciencedirect.com/science/article/pii/S0300483X14002133# This item was retrieved from the K-State Research Exchange (K-REx), the institutional repository of Kansas State University. K-REx is available at http://krex.ksu.edu Short-term oral atrazine exposure alters the plasma metabolome of male C57BL/6 mice and disrupts -linolenate, tryptophan, tyrosine and other major metabolic pathways Zhoumeng Lina,b,1, James R. Roedec,2, Chunla Hed, Dean P. Jonesc, Nikolay M. Filipova,b,* a Department of Physiology and Pharmacology, College of Veterinary Medicine, University of Georgia, Athens, GA 30602, USA; b Interdisciplinary Toxicology Program, University of Georgia, Athens, GA 30602, USA; c Division of Pulmonary, Allergy and Critical Care Medicine, Department of Medicine, Emory University, Atlanta, GA 30322, USA; d Department of Epidemiology and Biostatistics, College of Public Health, University of Georgia, Athens, GA 30602, USA Zhoumeng Lin: [email protected]; James R. Roede: [email protected]; Chunla He: [email protected]; Dean P. Jones: [email protected]; Nikolay M. Filipov: [email protected] * Corresponding author: Department of Physiology and Pharmacology, University of Georgia, 501 D. W. Brooks Dr., Athens, GA 30602, USA. Tel.: +1-706-542-3014; fax: +1-706-542-3015. E-mail address: [email protected] 1 Present address: Institute of Computational Comparative Medicine and The Department of Anatomy and Physiology, Kansas State University, Manhattan, KS 66506, USA. 2 Present address: Department of Pharmaceutical Sciences, Skaggs School of Pharmacy and Pharmaceutical Sciences, University of Colorado, Aurora, CO 80045, USA. Abbreviations: AE, anion exchange; ANOVA, analysis of variance; ATR, atrazine; C18, reverse phase; DACT, didealkylatrazine; DC-FTMS, dual chromatography-Fourier-transform mass spectrometry; DE, desethylatrazine; DIP, desisopropylatrazine; FDR, false discovery rate analysis; LOAEL, lowest observed adverse effect level; m/z, mass-to-charge ratio; PBPK, physiologically based pharmacokinetic; PCA, principal component analysis; PD, Parkinson’s disease; PUFAs, polyunsaturated fatty acids. 1 Abstract Overexposure to the commonly used herbicide atrazine (ATR) affects several organ systems, including the brain. Previously, we demonstrated that short-term oral ATR exposure causes behavioral deficits and dopaminergic and serotonergic dysfunction in the brains of mice. Using adult male C57BL/6 mice, the present study aimed to investigate effects of a 10-day oral ATR exposure (0, 5, 25, 125, or 250 mg/kg) on the mouse plasma metabolome and to determine metabolic pathways affected by ATR that may be reflective of ATR’s effects on the brain and useful to identify peripheral biomarkers of neurotoxicity. Four h after the last dosing on day 10, plasma was collected and analyzed with high-performance, dual chromatography-Fourier- transform mass spectrometry that was followed by biostatistical and bioinformatic analyses. ATR exposure (≥5 mg/kg) significantly altered plasma metabolite profile and resulted in a dose- dependent increase in the number of metabolites with ion intensities significantly different from the control group. Pathway analyses revealed that ATR exposure strongly correlated with and disrupted multiple metabolic pathways. Tyrosine, tryptophan, linoleic acid and α-linolenic acid metabolic pathways were among the affected pathways, with α-linolenic acid metabolism being affected to the greatest extent. Observed effects of ATR on plasma tyrosine and tryptophan metabolism may be reflective of the previously reported perturbations of brain dopamine and serotonin homeostasis, respectively. ATR-caused alterations in the plasma profile of α-linolenic acid metabolism are a potential novel and sensitive plasma biomarker of ATR effect and plasma metabolomics could be used to better assess the risks, including to the brain, associated with ATR overexposure. Keywords: Atrazine; Metabolomics; Biomarker; Pesticide; Metabolic pathway analysis; Dual chromatography-Fourier-transform mass spectrometry (DC-FTMS) 2 1. Introduction Atrazine (ATR) is a widely used herbicide and the most commonly detected pesticide in the groundwater, soil, and rain in agricultural areas of the US (Majewski et al. 2014; Toccalino et al. 2014). Groundwater ATR concentrations at levels close to the regulatory threshold value of 0.1 µg/L were reported 20 years after it was banned in Germany (Vonberg et al. 2014); 14C-labeled ATR could be detected in soil 22 years after application (Jablonowski et al. 2009), indicating environmental persistence and widespread potential for chronic, environmental exposures to low levels of ATR. Additionally, ATR exposure levels could reach up to 151,000 µg per work shift for ATR manufacturing workers (Catenacci et al. 1993) and 15.0 μg/m3 air for ATR applicators (Lozier et al. 2013), suggesting that the occupational exposure levels for ATR are much higher. Due to its widespread presence and continued use in most countries, including the US (EPA 2003), there is an increasing concern about ATR’s potential adverse health effects. In laboratory animals, excessive ATR exposure causes endocrine, reproductive, immune, and especially, nervous systems dysregulations (Cooper et al. 2007; Filipov et al. 2005; Lin et al. 2013a). For example, ATR exposure (50-300 mg/kg) decreases luteinizing hormone surge and disrupts estrous cycle in female rats (Cooper et al. 2007; Cooper et al. 1996). Chronic ATR (0.03-0.3 mg/kg) exposure also reduces basal metabolic rate, increases body weight and leads to insulin resistance in rats (Lim et al. 2009). Regarding its effects on the brain, we and others have shown in rodents that short-term (≥25 mg/kg), long-term (10 mg/kg), or perinatal (1.4 mg/kg) ATR exposures alter monoamine-associated behaviors and brain dopamine and serotonin homeostasis, suggesting that, in the brain, ATR targets tyrosine and tryptophan metabolism (Bardullas et al. 2011; Lin et al. 2013a; Lin et al. 2014). In line with the rodent studies, epidemiological data based on low, environmental ATR exposure levels report possible endocrine, i.e., menstrual cycle length irregularity (Cragin et al. 2011), metabolic, i.e., increased risk of gestational diabetes mellitus (Saldana et al. 2007), and neurologic, i.e., increased 3 incidence of Parkinson’s disease (PD; Shaw 2011) perturbations due to ATR. While laboratory and epidemiological data suggests that ATR overexposure is a potential risk factor for reproductive, metabolic and nervous system diseases, its exact mechanisms of toxicity and, importantly, reliable peripheral biomarkers of exposure and/or effect have not been elucidated. In order to shed light into ATR’s mode of action and aid the search for reliable biomarkers of ATR exposure, we developed physiologically based pharmacokinetic (PBPK) models for ATR in rodents of different ages (Lin et al. 2011; Lin et al. 2013b) that can be used for target organ dosimetry based on ATR’s peripheral biomarkers of exposure, i.e., plasma/urine levels of ATR and/or its metabolites, desethylatrazine (DE), desisopropylatrazine (DIP), and didealkylatrazine (DACT). We (Ross and Filipov 2006; Ross et al. 2009) and others (Barr et al. 2007; Chevrier et al. 2011; Fraites et al. 2011) have generated rodent and human data on the ATR’s kinetics and metabolite profile in plasma and/or urine that, with the help of PBPK modeling, can be used for ATR exposure dosimetry. However, at present, there are no mammalian data that can be used for peripheral biomarkers of ATR’s adverse effects. In an effort to discover peripheral biomarkers of toxic effects of environmental contaminants, researchers have begun to employ “metabolomics” approach in combination with bioinformatic and biostatistical methods. The term “metabolomics” can be defined as “the quantitative measurement of the dynamic multi-parametric metabolic response of living organisms to pathophysiological stimuli or genetic modification” (Nicholson et al. 1999). The advantage of this approach is that it allows rapid detection of both global changes of all metabolites or specific metabolites’ changes following exposure to xenobiotics using small amounts of easily accessible biological samples, such as blood or urine (Soltow et al. 2013). These altered metabolites can serve as biomarkers of toxicants’
Details
-
File Typepdf
-
Upload Time-
-
Content LanguagesEnglish
-
Upload UserAnonymous/Not logged-in
-
File Pages42 Page
-
File Size-