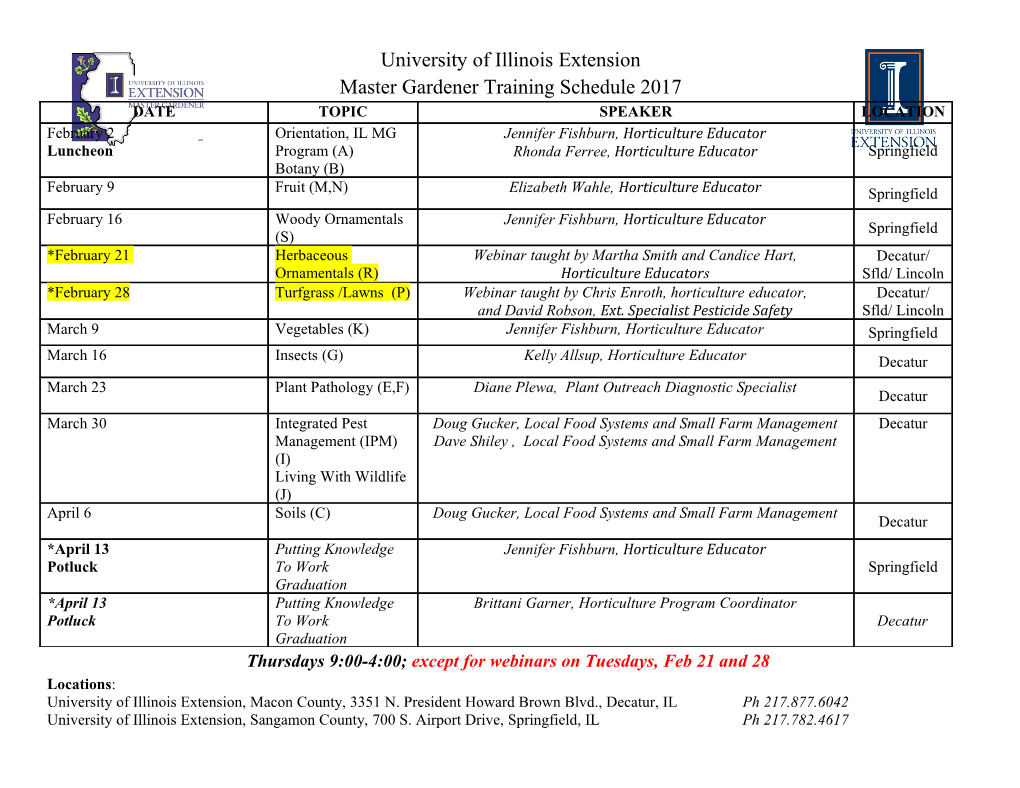
Astronomy & Astrophysics manuscript no. aanda ©ESO 2021 September 22, 2021 One star, two star, red star, blue star: an updated planetary nebula central star distance catalogue from Gaia EDR3 N. Chornay and N. A. Walton Institute of Astronomy, University of Cambridge, Madingley Road, Cambridge, CB3 0HA, United Kingdom e-mail: [email protected], e-mail: [email protected] September 2021 ABSTRACT Context. Planetary nebulae (PNe) are a brief but important phase of stellar evolution. The study of Galactic PNe has historically been hampered by uncertain distances, but the parallaxes of PN central stars (CSPNe) measured by Gaia are improving the situation. Aims. Gaia’s Early Data Release 3 (EDR3) offers higher astrometric precision and greater completeness compared to previous re- leases. Taking advantage of these improvements requires that the CSPNe in the catalogue be accurately identified. Methods. We applied our automated technique based on the likelihood ratio method to cross-match known PNe with sources in Gaia EDR3, using an empirically derived position and colour distribution to score candidate matches. Results. We present a catalogue of over 2000 sources in Gaia EDR3 that our method has identified as likely CSPNe or compact nebula detections. We show how the more precise parallaxes of these sources compare to previous PN statistical distances and introduce an approach to combining them to produce tighter distance constraints. We also discuss Gaia’s handling of close companions and bright nebulae. Conclusions. Gaia is unlocking new avenues for the study of PNe. The catalogue presented here will remain valid for the upcoming Gaia Data Release 3 (DR3) and thus provide a valuable resource for years to come. Key words. planetary nebulae: general – parallaxes – Methods: statistical 1. Introduction 2. Methods 2.1. Input catalogues Planetary nebulae (PNe) are a brief but enigmatic stage in the evolution of low- and intermediate-mass stars towards the ends Our PN positions come from the Hong Kong/AAO/Strasbourg of their lives. Central stars of PNe (CSPNe) are some of the rarest Hα (HASH) PN catalogue (Parker et al. 2016, as of 5 July 2021), objects observed by Gaia (Gaia Collaboration et al. 2016), a Eu- which now contains 2670 "true" PNe (those with spectroscopic ropean Space Agency (ESA) mission conducting precision as- confirmation; an 8% increase since CW20) and 3800 PNe in total (including likely and possible identifications). We saw in CW20 trometry and photometry for nearly two billion sources in the 00 Milky Way and beyond. Gaia shows great potential for the study that three very extended PNe had CSPNe more than 60 away of PNe: parallaxes inform distance estimates and in turn CSPN from the HASH positions, outside of our search radius. To ad- absolute magnitudes and evolutionary phase; proper motions add dress this we adopt positions for Sh 2-188 from Weidmann & more dimensions to dynamics and relate PNe to their parent stel- Gamen (2011) and for FP J1824-0319 and FP J0905-3033 from lar populations, constraining ages and masses; and repeated pho- Parker et al. (2006). Gaia EDR3 is likewise larger than DR2. tometric observations enable the study of variability and binarity. The longer survey duration (12 more months, for 34 months in total), along with improved calibration, results in higher com- pleteness (7% more sources overall) and greater astrometric pre- Unlocking Gaia’s potential requires a complete and accurate cision. The set of sources in EDR3 is distinct from that of DR2 cross-match of Gaia sources with CSPNe. Chornay & Walton not only in that more sources are present; some may have disap- (2020, hereinafter CW20) used an automated approach to gen- peared or changed identifiers (Torra et al. 2021). Gaia Data Re- erate an extensive catalogue of candidate CSPNe in Gaia Data arXiv:2102.13654v2 [astro-ph.SR] 21 Sep 2021 lease 3 (DR3) will include the same sources as EDR3 but with Release 2 (DR2, Gaia Collaboration et al. 2018). The nature additional data products. of Gaia’s iterative release process necessitates updates to cata- logues based on Gaia data, as the set of sources and their identi- fications changes between releases (Torra et al. 2021). Moreover, 2.2. Cross-matching when the Gaia data is itself used for cross-matching, better data translates into improved accuracy. In this work we present a new We use the same method employed in CW20, and here provide catalogue of candidate CSPNe and compact PNe in Gaia Early a brief review, pointing the reader to that work for a more de- Data Release 3 (EDR3, Gaia Collaboration et al. 2021) based on tailed background. The aim is to automatically match CSPNe the same methodology as CW20. With a completely new Gaia or compact/stellar-like nebulae to Gaia sources. The expectation catalogue not expected for some time, this will provide a valu- is that these sources will be close to the catalogued position of able resource for the study of Galactic PNe for years to come. the PN, but the possibility of non-detections, background inter- Article number, page 1 of 38 A&A proofs: manuscript no. aanda lopers, and positional inaccuracies necessitates a more nuanced approach than simple nearest-neighbour matching. The likelihood ratio method of Sutherland & Saunders (1992) is well-suited to this aim. Given a candidate match at angular separation r, the approach compares the probability of finding a genuine match at r to finding a background object. Our implementation assumes a uniform background density in each field, and a radially symmetric positional distribution of genuine CSPNe parameterised by the radius of the PN (allowing larger PNe to have more offset CSPNe, albeit with lower probability). We also employ the colour distributions of true CSPNe and back- ground sources, parameterised based on the photometric excess factor (Evans et al. 2018), with the effect of down-weighting colours for sources whose colour is potentially contaminated by nearby sources or background. Distributions are derived itera- tively, using sources with secure identifications based on colour to empirically determine the positional distribution of genuine CSPNe, and in turn using the positionally secure sources to up- date the colour distribution. This works because CSPNe are of- Fig. 1: Matching results for "true" PNe. The top left panel shows ten much bluer than typical Gaia sources and therefore can be the reliability of the CW20 Gaia DR2 matches versus the EDR3 selected purely based on colour with relatively high confidence. matches from this work for PNe present in both. Red points are We made a few small changes to the algorithm’s treatment PNe where the location of the best candidate match has changed 00 of the GBP–GRP colours. In deriving the initial and final colour by more than 0:1. All of these points are contained in the his- distributions, we only considered sources with G brighter than togram in the lower left panel, which also includes PNe that were 19, which have relatively precise colours. Then in computing the not in CW20. The upper right panel shows the separation versus likelihood ratios for individual sources we convolved the densi- colour distribution of highest-ranked candidates, colour-coded ties with the photometric uncertainties, after accounting for the by reliability with the non-linear scale shown at the bottom right. smoothing already applied by the kernel density estimation. This Rings around markers indicate PN angular sizes. lessens the impact of faint sources with extreme and likely spu- rious colours. We also adopted the prescription of Riello et al. Table 1: Counts of PNe occupying different regions of the scat- (2021) for describing a locus of photometric excess values of ter plot in the upper left corner of Fig. 1, representing different well-behaved sources. The photometric excess is no longer used changes in reliability relative to the CW20 Gaia DR2 matches. by Gaia as a filter for published photometry, though for large ex- cess values the photometry is still not very discriminative, as it PN status match location region likely dominated by the background. 00 ABCDE We retrieved all Gaia EDR3 sources within a 60 radius of true same 53 105 1238 47 15 the PN positions from HASH. All of these were used in calcu- changed 16 14 10 14 157 lating the background density and colour distribution (the latter other same 36 58 303 7 3 only for G < 19), while only the subset of sources within half changed 3 3 2 4 30 each PN angular size (taken from HASH) plus 200 were consid- ered as candidates. For each PN, the set of candidate likelihood ratios were used to calculate reliabilities (the probability that a given candidate is the correct match). The complete catalogue of the 2117 highest ranked candi- dates with reliability > 0.2 is in Table A.1. The catalogue in- cludes the Gaia source identifier corresponding to the best match 3. Catalogue for each PN, the reliability of that match, and its angular separa- tion from the PN position from HASH. Copied into the catalogue The improved method applied to EDR3 produces a bimodal dis- are relevant data from HASH (name, PN G identifier, PN coor- tribution of top match reliabilities similar to that from CW20 dinates, angular size, and confirmation status) and Gaia EDR3 for Gaia DR2 (Fig 1, lower left; for clarity only "true" PNe are (source coordinates, photometry, and astrometry). We addition- shown). Most sources have reliabilities close to their values from ally include the image parameter determination (IPD, Lindegren CW20; these are near the diagonal (region C) in upper left of et al. 2021b) goodness-of-fit harmonic amplitude from Gaia (see Fig. 1.
Details
-
File Typepdf
-
Upload Time-
-
Content LanguagesEnglish
-
Upload UserAnonymous/Not logged-in
-
File Pages38 Page
-
File Size-