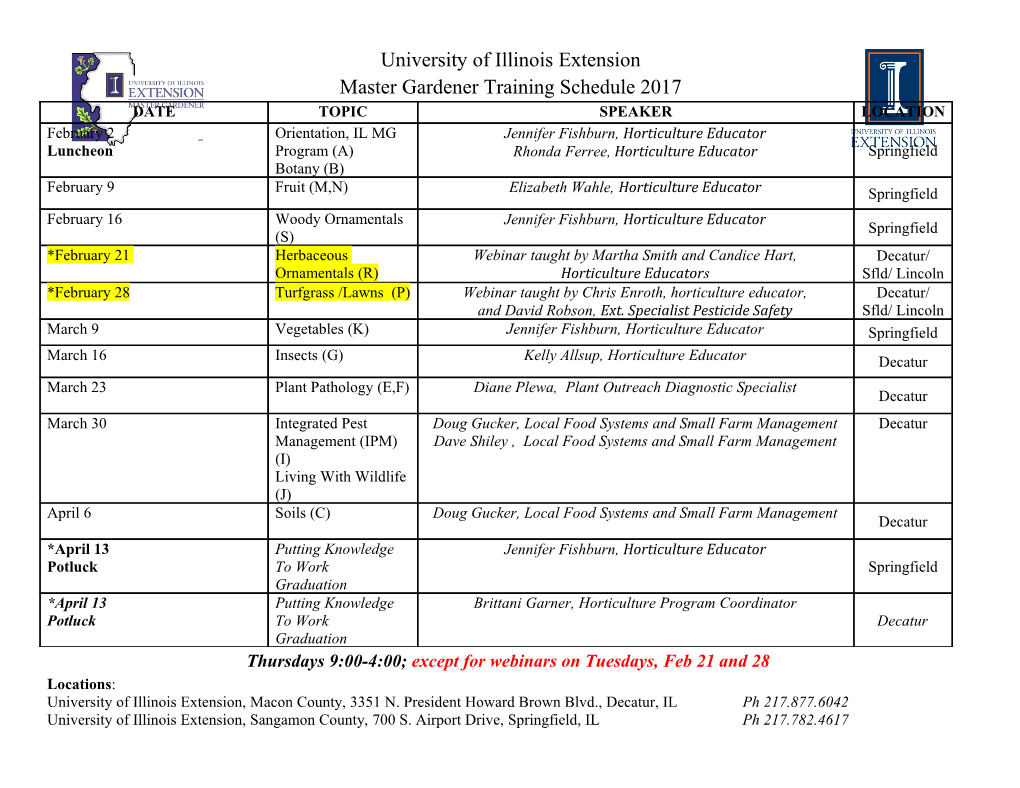
REDUCTIVE ELIMINATION OF ALKYLAMINES AND ETHERS: REACTIONS OF BISPHOSPHINE-LIGATED PALLADIUM(II) COMPLEXES BY SETH L. MARQUARD DISSERTATION Submitted in partial fulfillment of the requirements for the degree of Doctor of Philosophy in Chemistry in the Graduate College of the University of Illinois at Urbana-Champaign 2011 Urbana, Illinois Doctoral committee: Professor John F. Hartwig, Chair Professor Thomas B. Rauchfuss Professor Patricia A. Shapley Professor M. Christina White Abstract The reductive elimination reactions detailed in this dissertation provide experimental insight into the mechanism of reductive elimination to form the C(sp3)-N bond of benzylamines and the C(sp3)-O bond of benzyl ethers. The stereochemical outcome of the reaction indicates an ionic pathway, but the process lacks many of the effects of electronic and solvent perturbations that typically signal an ionic intermediate. We propose that reductive elimination from benzylpalladium(II) amido and aryloxide complexes occurs by dissociation of the amido or aryloxide ligand, followed by nucleophilic attack on the benzyl ligand. The proposed ionic mechanism is more akin to the reductive elimination reactions that occur from high-valent Pt(IV) and Ni(III) complexes than reductive elimination reactions that occur from other Pd(II) complexes. Our data indicate that substantial differences exist between reductive eliminations to form the C(sp3) bonds in ethers and amines from palladium(II). We prepared alkylpalladium(II) amido complexes to study the C(sp3)-N reductive elimination reaction from complexes containing a non-benzylic hydrocarbyl ligand. We investigated a series of alkylpalladium amido complexes and observed reductive elimination occurs from bisphosphine-ligated neopentylpalladium amido complexes in low yield. Reductive elimination from neopentylpalladium amido complexes occurs most likely by a concerted reductive elimination reaction, and is favored by the increased steric bulk of the neopentyl ligand. We also investigated azametallacyclic palladium complexes with a norbornyl hydrocarbyl ligand, and observed reductive elimination occurs to form a norbornyl indoline product. We found that the yield was slightly improved over neopentylpalladium complexes, but that the yield of reductive elimination was low. Finally we investigated non-metallacyclic complexes containing a norbornyl hydrocarbyl ligand. We discovered that reductive elimination ii occurs in moderate yield, and the reductive elimination product ratio indicates a balance between a concerted and an ionic mechanism. The data presented in this dissertation demonstrate that C(sp3)-N reductive elimination from benzylpalladium(II) and alkylpalladium(II) complexes can occur. We propose an ionic mechanism for the formation of benzylamines and benzyl ethers by reductive elimination from benzylpalladium(II) complexes. Reductive elimination from neopentyl and metallacyclicpalladium(II) complexes likely occurs by a concerted mechanism, demonstrating the importance of steric bulk and metal geometry, respectively. Finally, reductive elimination from non-metallacyclic norbornylpalladium(II) complexes indicates that a concerted and ionic mechanism may occur simultaneously. Although the yield of the alkylamine products is low, the observation that C(sp3)-N reductive elimination occurs from the alkylpalladium complexes provides the first step toward developing a synthetically useful reaction for the formation of C(sp3)-heteroatom bonds from low-valent group 10 complexes without the addition of an oxidant. iii Acknowledgements Graduate school has taught me many valuable life lessons that I would not have gained by any other method. I would like to thank all of the people who have helped me grow and develop both as a chemist and as a person. This journey has been one of the most challenging experiences in my life thus far, and I couldn’t have done it alone. Gratitude is extended to thank Jackie Murphy-Knight and Jesse Tye for their continued friendship and for their humor and wit throughout. I would like to thank Eric Simmons and Levi Stanley for always being available to confer about organic chemistry. I would also like to thank my fellow classmates Chris Letko and Matt Thorseth for the friendship and entertainment outside of lab. Finally, I would like to express my gratitude to my fellow lab mate Cass Richers for the years of late-night hijinks. I would like to thank my advisor John Hartwig for educating me in all aspects of chemical research. John always expects the best from his students, and living up to those high expectations is not always an easy task. Under his tutelage I learned an incredible amount about conducting research, designing experiments, and thinking critically. Thanks are extended to him for his time and effort that shaped me into a better chemist. Of course I would be remiss to leave my amazing parents off the list of people who have helped me throughout this experience. They instilled in me a hard-work ethic and a desire to explore the world around me. Memories of helping my father in his lab as a boy are still fresh in my mind, and that experience laid an important foundation for my scientific life. Lastly, I would like to thank Amanda Mack for providing me with some of the happiest times of my life, and for helping me through all the challenges and hurdles associated with graduate school. Thank you to everyone. iv Table of Contents Chapter 1: Reductive Elimination: Parameters that Influence the Relative Rate, and Examples of C-Heteroatom Reductive Elimination Reactions…………...……….1 Chapter 2: C(sp3)-N Bond-Forming Reductive Elimination of Amines. Reactions of Bisphosphine-Ligated Benzylpalladium(II) Diarylamido Complexes………......61 Chapter 3: C(sp3)-O Bond-Forming Reductive Elimination of Ethers. Reactions of Bisphosphine-Ligated Benzylpalladium(II) Aryloxide Complexes……………127 Chapter 4: C(sp3)-N Bond-Forming Reductive Elimination of Alkylamines. Reactions of Bisphosphine-Ligated Alkylpalladium(II) Amido Complexes…………………174 v Chapter 1: Reductive Elimination: Parameters that Influence the Relative Rate, and Examples of C-Heteroatom Reductive Elimination Reactions. ______________________________________________________________________________ 1.1 Introduction to Reductive Elimination Organometallic chemistry is dominated by a handful of fundamental bond-forming or bond-breaking transformations: oxidative addition, reductive elimination, migratory insertion, transmetalation, and β-hydride elimination. Catalytic reactions are composed of several of these elementary reactions, and the elementary reaction of reductive elimination is the product-release step of many catalytic cycles. While mild catalytic methods for the formation of carbon-carbon bonds have been well developed, comparable mild catalytic methods for carbon-heteroatom bond-forming reactions were only recently developed. The ability to form carbon-heteroatom bonds by a catalytic process could provide a powerful strategy for fine and commodity chemical synthesis.1, 2 The elementary reductive elimination reaction forges a bond between two anionic metal- bound ligands and results in a reduction of the metal’s formal oxidation state by two units. A generic reductive elimination reaction is shown in Equation 1.01. Both carbon-carbon and carbon-heteroatom bonds can be formed by reductive elimination. These reactions serve as the basis for Suzuki, Negishi, Kumada, Sonogashira, Buchwald-Hartwig, Chan-Lam, and Ullmann cross-coupling reactions.1, 2 Numerous factors, including orbital symmetry, bite angle, coordination number, oxidation state, and steric effects, influence the rate of reductive elimination. These parameters will be discussed in depth individually in the following sections. 1 1.2 Factors that Affect the Rate of Reductive Elimination 1.2.1 Orbital Symmetry Square planar complexes serve as intermediates in catalytic transformations such as Suzuki, Negishi, Kumada, Sonogashira, or Buchwald-Hartwig cross-coupling reactions. The parameters that affect the relative rate of reductive elimination from d8 square planar complexes have been studied computationally for catalytically relevant organometallic complexes of the 3 general type (A)2M(R)2, in which A = PH3, H; M = Ni, Pd; R = H, CH3. A schematic orbital correlation diagram for reductive elimination from a cis-(A)2M(R)2 complex to form (A)2M with expulsion of R-R is shown in Figure 1.01. The orbital of interest is the low-lying 1b2 orbital, which increases in energy and becomes metal-centered during the reductive elimination reaction. The difference in energy between the ground-state energy level of the 1b2 orbital and the metal- centered b2 orbital dominates the activation energy for the reductive elimination. Factors that stabilize the b2 orbital inhibit reductive elimination, which is the case for nickel complexes due to the lower-energy d-orbitals of nickel relative to palladium. The energy level of the b2 orbital can also be affected by the donating ability of the ligands. Strong σ-donor reacting ligands (R) raise the relative energy of the ground-state configuration, which accelerates the rate of reductive elimination because the activation energy is diminished. Strong σ-donating ancillary ligands (A) raise the relative energy of the metal-containing product, thus raising the activation energy. 2 y R-R x b2 = a1 a2 b1 = = = = = = = = a1 b2 M-R = = a1 R-R = L L L L M M +R-R R R C 2v Figure 1.01 Orbital correlation diagram for d8 square planar complexes undergoing
Details
-
File Typepdf
-
Upload Time-
-
Content LanguagesEnglish
-
Upload UserAnonymous/Not logged-in
-
File Pages227 Page
-
File Size-