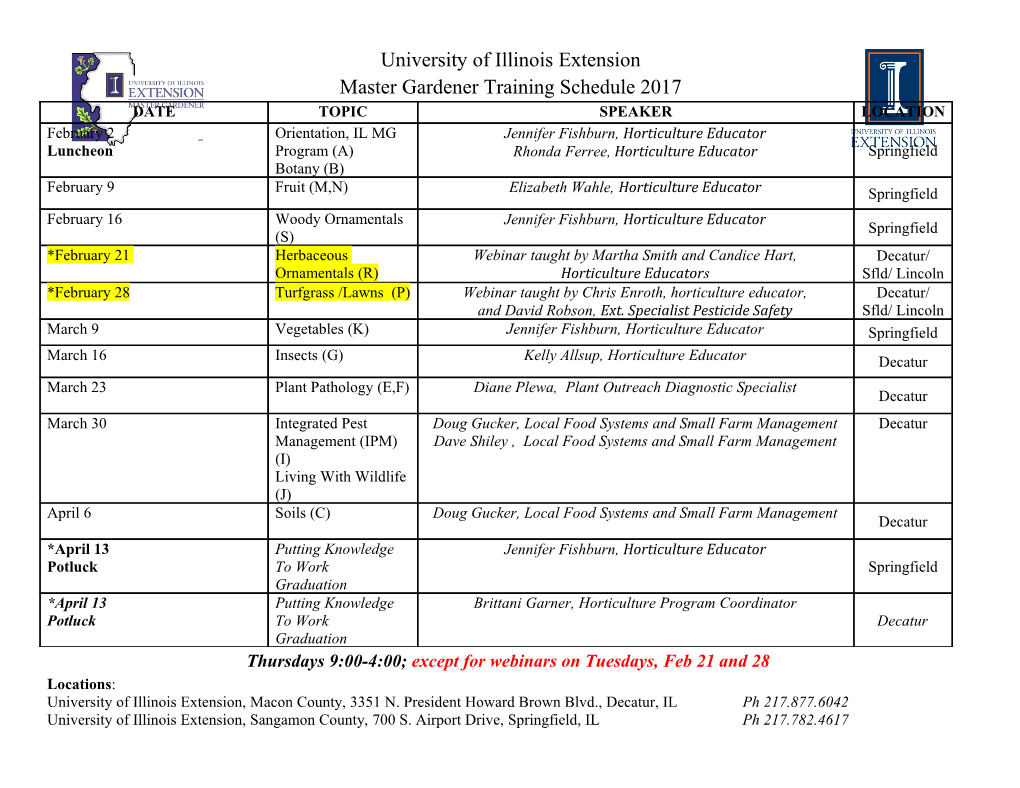
MUSCLE BIOCHEMISTRY Biochemistry of Lean Muscle Tissue as Related to Water-Holding Capacity Riette L.J.M. van Laack* Morse B. Solomon Introduction (muscle cells) bounded by a plasma cell membrane, sur- rounded by a thin connective tissue network, the endomysium. The literature on water-holding contains many references Each contractile fibre is composed of myofibrils 1 to 2 pm in to the term “water-holding capacity.” Water-holding capacity diameter. These myofibrils are separated from one another (WHC) has been defined as the ability of meat to retain its by spaces containing sarcoplasmic reticulum and mitochon- own water despite the application of force. We will use the dria. Myofibrils consist of contractile filaments ordered in a term WHC as it relates to drip losses from fresh uncooked regular way; this arrangement is responsible for the striated meat. Drip loss and WHC will be used as alternative terms for appearance of skeletal muscle. The smallest contractile unit, the same phenomenon. a sarcomere, consists of thick myosin filaments, thin actin fila- The amount of drip lost from carcasses is negligible, but ments and a network of longitudinal and transverse elements; after breaking, drip losses are of the order of 0.1 to 1 Yo during the cytoskeletal network. 2 days. When the meat is further fabricated (steaks etc.), drip losses increase and may exceed 10%. Water in the Muscle: Water-holding is of great importance, because moisture How Much, Where? losses affect the weight and therefore the financial value of the meat. Moreover, exudation of drip produces an unsightly From the point of view of quantity, water is the most impor- pool of liquid around the meat which adversely affects the tant constituent of meat. At the time of slaughter, muscle con- appearance and consumer appeal. As drip has about two- tains about 75% water. Hamm (1975) estimated that approxi- thirds the protein concentration of whole meat, its loss is a mately 85% of this water is located intracellularly, primarily in costly waste of animal protein. The content of water and its the spaces between thick and thin filaments, but also in the distribution have a profound influence on the sensory proper- sarcoplasm and in connective tissue. The remaining 15% is ties of meat. located in extracellular spaces. According to Hamm (1972), Information on the mechanisms responsible for drip for- the different muscle components contribute to water-holding mation will provide better means of controlling and predicting capacity as follows: myofibrillar proteins 50%, sarcoplasmic WHC. Also, knowledge of the processes involved may allow proteins 3%, non-protein components of sarcoplasma 47%. for the prediction of the impact of new technologies on this A portion of water (4 to 1Og/l OOg protein) is bound very quality attribute. tightly. A much larger amount, 20 to 60gil OOg protein, of wa- In the following, we give a short overview of the current ter is located in a less organized fashion between the protein knowledge on WHC and indicate which aspects need more molecules. This loosely (electrostatically) bound water is af- attention. It was not the purpose of the authors to provide an fected by molecular changes brought about by protein dena- extensive review. For more detailed information, the reader is turation. referred to reviews by Offer and Knight (1988) and Hamm Since proteins form about one-fifth of the wet-weight of (1 972; 1986). meat, and the weight 3f bound water is about half the weight of the protein (1 Og), water bound (firmly or loosely) to the pro- Muscle Structure tein in meat amounts maximally to about 10% of the muscle weight. The remaining water (70%) must be held within the A muscle is completely enclosed by a sheath of connec- meat structure by capillary forces. Since myofibrils occupy ap- tive tissue, the epimysium. Muscle is divided into bundles of proximately 70% of the volume of lean muscle, most of the fibres by a connective tissue network, the perimysium. The tissue water must be located in the myofibrils. The myofibrils muscle bundles, in turn, consist of long, contractile fibres are well suited to retain water because of the three-dimen- sional network of the filaments. The amount of water immobi- lized depends on the space available between the filaments. *R.L. J. M. van Laack, USDA, Agricultural Research Service, Beltsville, MD 20705. The Origin of Drip Losses M.B. Solomon, USDA, Agricultural Research Service, Considering the amount of water bound to proteins and Beltsville, MD 20705. the amount of water lost from meat, it appears unlikely that WHC is related to the bound water. Any large changes in the Reciprocal Meat Conference Proceedings, Volume distribution of water within the meat structure must, by neces- 47, 1994. sity, originate from changes in myofilament structure. Swell- 91 92 American Meat Science Association ing or shrinkage of the muscle fibre is caused by expansion or Figure 1 shrinkage of the myofilament lattice and the resultant water movement between intracellular and extracellular spaces. It should be emphasized that swelling or shrinkage of myofibrils alters the distribution of water within the muscle but does not necessarily affect the volume of the muscle as a whole (Offer and Knight, 1989; Fig. 1). (a) The hypothesis that WHC is determined by myofibrillar shrinkage and movement of water from the myofilament space into the sarcoplasmic space and subsequently into extra- cel- lular space is supported by experimental data: NMR: H-pulse-NMR is a powerful, non-invasive tool for studying water in tissues. Water protons in (b) muscle have a shorter transverse relaxation time than water protons in bulk water. A long relax- ation time suggests a long distance of the free water protons to the site where these can ex- change with hydration water. This means that larger "pockets" of water within the structure have a greater chance of obtaining relaxation times similar to that of free water than water in small (C) pores. Post-rigor, there is a larger percentage of water with long (100-1 50ms) relaxation times, in- dicating more water in large pores (extracellular spaces) than in pre-rigor meat (Renou et al., 1985; Fjelkner and Tornberg, 1986; Tornberg et at., 1993). Fibre Diameter Measurements with Light Microscopy (d) These indicate that the pre-rigor fibre diameter is larger than the post-rigor, e.g. the post-rigor myofibrils are shrunk (Offer and Knight, 1988). Using light microscopy, Penny (1977) and Larsson and Tornberg (1988) observed a larger extracel- lular volume for PSE (Pale Soft Exudative) meat than for normal meat. Diesbourg et al. (1988) demonstrated that the distance between filaments, measured with X-ray diffraction, becomes smaller during rigor onset, indicater shrinkage of fibre di- ameter. Measurements with Inulin The extracellular space can be studied by in- The relationship between fibre volume and muscle volume; (a) to (e) cubating a thin muscle with non-metabolic sug- show varying degrees of swelling and shrinkage. ars, such as inulin, which are presumed not to Offer and Knight, 1989. enter the muscle. After equilibration, the amount of the substance in the muscle gives an indica- et al. (1989) suggested the following path for drip loss from tion of the size of the extracellular space. Currie the muscle: and Wolfe (1 980; 1983) showed that the post-rigor the myofilament lattice muscle takes up more inulin than pre-rigor muscle, 1 indicating that post-rigor muscle has a larger ex- the interfibrillar sarcoplasm tracellular compartment than pre-rigor muscle. 1 the interfibre space Offer and Cousins (1992) have shown that fibre and fibre 1 bundles shrink post-mortem when their constituent myofibrils the interfascicular space shrink, thus resulting in the formation of two extracellular com- 1 partments around the fibres and the fibre bundles. Swatland drip loss 47th Reciprocal Meat Conference 93 Offer et al. (1989) confirmed that the drip arises predomi- Protein Denaturation nantly from the longitudinal channels through the meat be- tween the fibre bundles. The main force for drip formation Approximately 95% of total muscle protein is located within seems to be gravity. the myofibre. Depending on their nature and function, intra- Presuming that myofibrillar shrinkage (the interfilament cellular proteins can be classified as sarcoplasmic proteins or myofibrillar proteins. Sarcoplasmic proteins are dissolved in spacing) is indeed the main determinant of WHC, we have to the sarcoplasm, and play an important role in cellular metabo- focus on the factors that may affect myofibrillar shrinkage. There are three main factors involved in the shrinkage and/or lism. These proteins are soluble in low ionic strength solu- swelling of myofibrils: tions. Myofibrillar proteins are insoluble in water or low ionic strength solutions. 1. onset of rigor mortis 2. the extent of pH decline A protein in an aqueous solution interacts with water. Part 3. protein denaturation of this water is electrostatically bonded to the surface of the a. myosin denaturation molecule and part may be entrapped. Denaturationof the pro- b. sarcoplasmic protein denaturation tein will affect the structure and charge of the protein and there- Each of these factors will be discussed in the following para- fore the binding of water and solubility of the protein. graphs. Myosin Denaturation Onset of Rigor Mortis There is good evidence that myosin is involved in WHC; At rigor onset, there is a rapid decline (about 4.4%) in fila- specifically in the loss of WHC in PSE meat. ment spacing due to attachment of myosin heads to actin (Of- Salt solubility of myofibrillar proteins and ATP- fer et al. 1989). The amount of shrinkage will be smaller at ase activity are lower in PSE meat than in normal longer sarcomeres (see sarcomere length).
Details
-
File Typepdf
-
Upload Time-
-
Content LanguagesEnglish
-
Upload UserAnonymous/Not logged-in
-
File Pages7 Page
-
File Size-