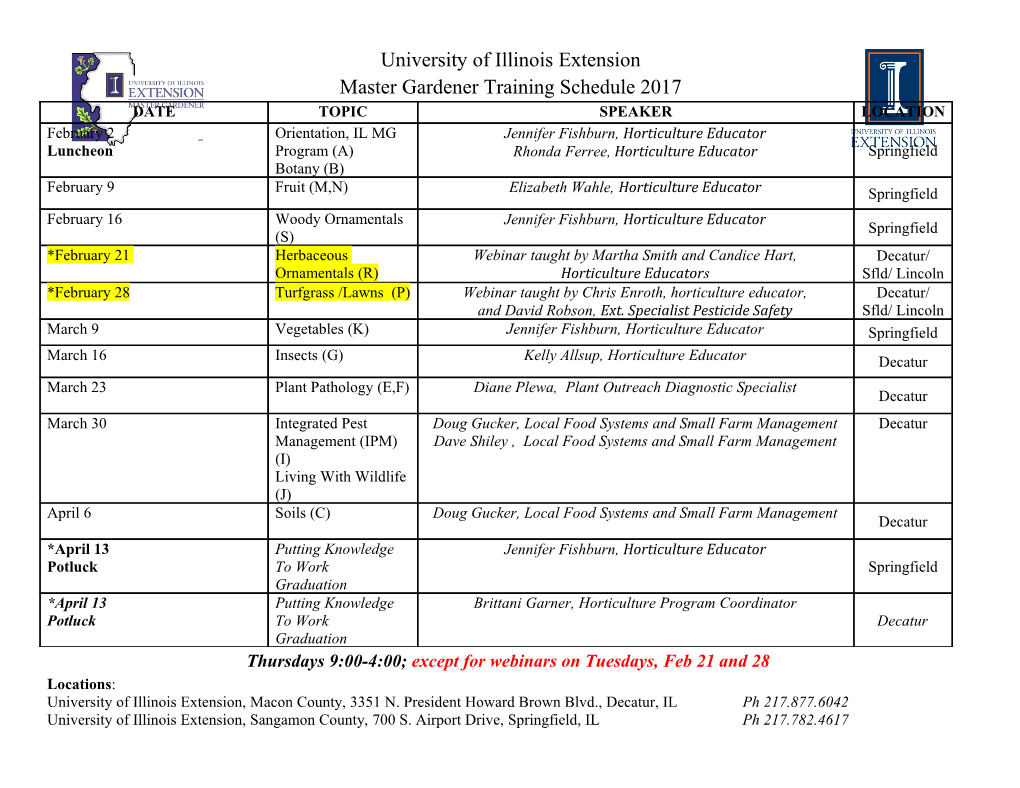
VU Research Portal Regulation of neuronal plasticity by the transcription factor NFIL3 van der Kallen, L.R. 2016 document version Publisher's PDF, also known as Version of record Link to publication in VU Research Portal citation for published version (APA) van der Kallen, L. R. (2016). Regulation of neuronal plasticity by the transcription factor NFIL3. General rights Copyright and moral rights for the publications made accessible in the public portal are retained by the authors and/or other copyright owners and it is a condition of accessing publications that users recognise and abide by the legal requirements associated with these rights. • Users may download and print one copy of any publication from the public portal for the purpose of private study or research. • You may not further distribute the material or use it for any profit-making activity or commercial gain • You may freely distribute the URL identifying the publication in the public portal ? Take down policy If you believe that this document breaches copyright please contact us providing details, and we will remove access to the work immediately and investigate your claim. E-mail address: [email protected] Download date: 27. Sep. 2021 Regulation of neuronal plasticity by the transcription factor NFIL3 Loek van der Kallen The research described in this thesis was performed at the department of Molecular and Cellular Neurobiology, Center for Neurogenomics and Cognitive Research, Neuroscience Campus Amsterdam, VU University, Amsterdam, The Netherlands. Cover by Loek van der Kallen VRIJE UNIVERSITEIT Regulation of neuronal plasticity by the transcription factor NFIL3 ACADEMISCH PROEFSCHRIFT ter verkrijging van de graad Doctor aan de Vrije Universiteit Amsterdam, op gezag van de rector magnificus prof.dr. V. Subramaniam, in het openbaar te verdedigen ten overstaan van de promotiecommissie van de Faculteit der Aard- en Levenswetenschappen op dinsdag 2 februari om 15.45 uur in de aula van de universiteit, De Boelelaan 1105 door Loek van der Kallen Geboren te Utrecht promotoren: prof.dr. A.B. Smit prof.dr. J Verhaagen copromotor: dr. R.E. van Kesteren The knack of flying is learning how to throw yourself at the ground and miss Douglas Adams Leescommissie: prof.dr. M.P. Smidt prof.dr. S. Spijker dr. H.D. MacGillavry dr. K. Bossers dr. A.J.A. Groffen Contents Chapter 1 ................................................................................................................ General introduction ........................................................................................ 9 Chapter 2 ................................................................................................................ Genetic deletion of the transcriptional repressor NFIL3 enhances axon growth in vitro but not axonal repair in vivo .................................................. 29 Chapter 3 ................................................................................................................ Behavioral characterization of Nfil3 knockout mice ...................................... 57 Chapter 4 ................................................................................................................ Behavioral inflexibility in mice lacking the transcriptional repressor NFIL3 .. 71 Chapter 5 ................................................................................................................ CAGE sequencing reveals aberrant immediate-early gene expression during memory reconsolidation in Nfil3 knockout mice ........................................... 87 Chapter 6 ................................................................................................................ General discussion ........................................................................................ 107 English summary .............................................................................................. 119 References ........................................................................................................ 125 Acknowledgements .......................................................................................... 143 About the author .............................................................................................. 151 Chapter 1 General introduction Chapter 1 Neurons retain a level of plasticity in order to adapt to external needs and stimuli throughout adult life. This plasticity occurs at the level of neuronal structure and function. In particular, plasticity of neurons can be observed at their numerous points of contact, the synapses. These synapses are built of axonal pre- and dendritic postsynaptic elements (spines). At the structural level, for instance, a continuous turnover of dendritic spines is observed in many brain areas, which affects synapse density and can be modified by neuronal activity [1], aging [2], in diseases, such as schizophrenia [3] and fragile-x syndrome [4], and during and after drug use [5]. Dendrite morphology can also be affected, for instance, by stress [6,7], in Alzheimer’s [8] and Huntington’s disease [9], fragile-x syndrome [4] and after drug use [5]. Axons also show variation in length and arborisation throughout adulthood, both under normal conditions [10,11] and, for instance, after diverse forms of brain injury [12]. Thus, dendritic and axonal plasticity enable synaptogenesis, i.e. the creation of new synapses, whenever this is required [13,14]. More specifically, an increase in synapse densities results from differences in the rates of spine generation, stabilization and elimination [15], processes that persist into adulthood [14]. In addition to apparent structural plasticity, in the CNS other forms of plasticity are found. Modulation of the firing strength of existing synapses occurs, both pre- [16,17] and postsynaptically [18,19], and either directly, or indirectly through signals from glia [20]. Collectively, all these different forms of neuronal plasticity enable changes at a circuitry level that allow individuals to optimally cope with a continuously changing environment. Forms of plasticity that occur over longer timescales, e.g. 2 hours and more, require de novo gene transcription and protein synthesis [21,22,23]. Transcription factors that regulate the transcriptional response to diverse forms of stimulation are key elements in the signaling pathways leading to long-term neuronal plasticity. In this chapter, I will discuss the role of transcription factors in various forms of neuronal plasticity. Specifically, I will introduce two forms of plasticity; regenerative plasticity following peripheral nerve injury and synaptic plasticity underlying cognitive processing, and the role of gene expression in 10 General introduction each. Subsequently, I will discuss transcriptional regulation and gene regulatory networks in more detail, and introduce the two transcription factors that are the main focus of this thesis. Finally, I will briefly introduce the techniques that were used in this thesis to study neuronal regeneration, cognition and transcriptional regulation. Neural plasticity and the regeneration of injured axons Regenerating neurons show a large degree of plasticity. Damaged axons need to grow new arbors in appropriate directions, identify and connect with appropriate targets, and fine-tune their firing strength. When lesions occur in the central nervous system (CNS), for instance the spinal cord, during adult life, axons will not regenerate, likely due to non-permissive signals expressed by oligodendrocytes and glial scar tissue, and the debilitating effects are enormous [24,25]. However, when a nerve lesion occurs in the peripheral nervous system (PNS), axons are better capable of entering a state of plasticity and growth. One of the main differences between the CNS and the PNS environment is that PNS axons are not myelinated by oligodendrocytes but by Schwann cells, which provide a more permissive environment for regenerative axon growth. The growth-promoting properties of Schwann cells have been exploited for instance to generate permissive nerve grafts for spinal cord injury sites [26,27]. In human patients, autologous Schwann cells have been transplanted into spinal cord injury sites during clinical trials with results ranging from no adverse effects [28] to positive effects on motor and sensory functions [29]. Another cell type that is efficient at inducing a regenerative growth state in neurons is olfactory ensheathing cells, which are currently also used in clinical trials with some cautiously promising results [30]. The regenerative plasticity of a neuron is in part determined intrinsically, as was demonstrated by the so-called conditioning lesion paradigm, where a CNS injury shows better regeneration when preceded by a PNS lesion [31]. Three types of injury signals together prepare and induce the cell body to respond to the specific needs of axonal regeneration [32]. Firstly, a 11 Chapter 1 rapid influx of calcium and sodium induces depolarization and a train of action potentials, resulting in swelling of the neuronal cell body and a strong increase in cellular metabolism and protein synthesis (Fig. 1.1) [33]. Secondly, disruption of molecular transport from the originally innervated target prevents retrograde flow of negative regulators of plasticity (Fig. 1.2) [34]. Thirdly, positive injury signals, including proteins derived from neighboring axons and glia, but also locally translated proteins and activated kinases, induce plasticity in the cell body (Fig.
Details
-
File Typepdf
-
Upload Time-
-
Content LanguagesEnglish
-
Upload UserAnonymous/Not logged-in
-
File Pages153 Page
-
File Size-