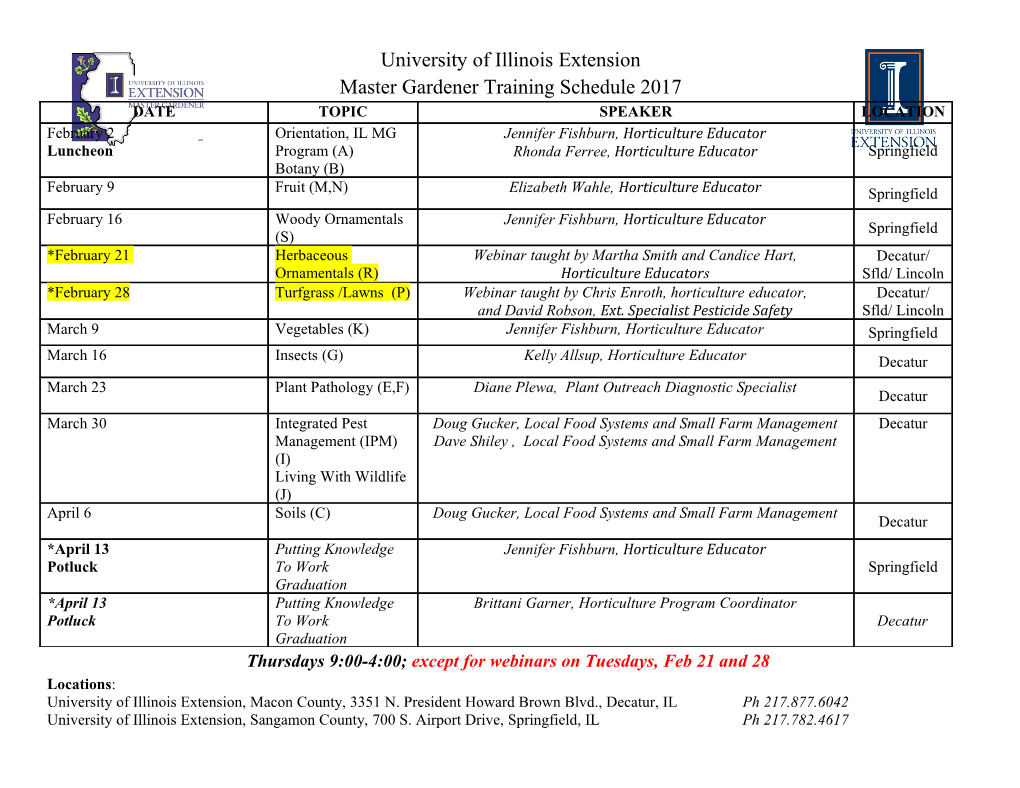
Glacial ocean circulation and stratification explained by reduced atmospheric temperature Malte F. Jansena,1 aDepartment of the Geophysical Sciences, The University of Chicago, Chicago, IL 60637 Edited by Mark H. Thiemens, University of California, San Diego, La Jolla, CA, and approved November 7, 2016 (received for review June 27, 2016) Earth’s climate has undergone dramatic shifts between glacial and We test the connection between atmospheric temperature interglacial time periods, with high-latitude temperature changes and ocean circulation and stratification changes, using idealized on the order of 5–10 ◦C. These climatic shifts have been asso- numerical simulations, which allow us to isolate the proposed ciated with major rearrangements in the deep ocean circulation mechanism. We use a coupled ocean–sea-ice model, with atmo- and stratification, which have likely played an important role in spheric temperature, winds, and evaporation–precipitation pre- the observed atmospheric carbon dioxide swings by affecting the scribed as boundary conditions (Materials and Methods). The partitioning of carbon between the atmosphere and the ocean. model uses an idealized continental configuration resembling the The mechanisms by which the deep ocean circulation changed, Atlantic and Southern Oceans, where the most elemental circu- however, are still unclear and represent a major challenge to our lation changes have been inferred (3, 5, 6, 12). understanding of glacial climates. This study shows that vari- ous inferred changes in the deep ocean circulation and stratifica- Results tion between glacial and interglacial climates can be interpreted We first focus on the model’s ability to reproduce key features as a direct consequence of atmospheric temperature differences. of the modern (interglacial) ocean state and circulation. Fig. 1A Colder atmospheric temperatures lead to increased sea ice cover shows the sea surface temperature (SST) over the simu- and formation rate around Antarctica. The associated enhanced lated domain, with boundary conditions resembling present-day brine rejection leads to a strongly increased deep ocean strati- atmospheric forcing. The chosen forcing gives SSTs in broad fication, consistent with high abyssal salinities inferred for the agreement with observations, varying from about 28◦C in the last glacial maximum. The increased stratification goes together tropics to the freezing point (∼ −2 ◦C) around Antarctica, where with a weakening and shoaling of the interhemispheric over- sea ice forms (Fig. 1A, white contours). The North Atlantic is turning circulation, again consistent with proxy evidence for the somewhat warmer and ice free. Fig. 2 shows zonally averaged last glacial. The shallower interhemispheric overturning circula- depth–latitude sections of potential temperature (Fig. 2A) and tion makes room for slowly moving water of Antarctic origin, salinity (Fig. 2C), which reproduce the basic features observed which explains the observed middepth radiocarbon age maximum in the present-day Atlantic. In particular, we see a tongue of and may play an important role in ocean carbon storage. salty water at middepth, penetrating southward into the South- ern Ocean and leading to a reversal of the salinity stratifica- LGM j AMOC j stratification j cooling j sea-ice tion in the abyss (2). The associated overturning streamfunc- tion (Fig. 3A) reveals that this salty tongue is associated with NADW that flows southward as part of the Atlantic meridional he deep ocean today is ventilated mainly by two water overturning circulation (AMOC). Below the clockwise AMOC masses. North Atlantic deep water (NADW) is formed in T cell lies an anticlockwise abyssal cell, representing the pathway the North Atlantic before flowing southward at a depth of about of AABW. The peak overturning transports of +17.5 Sverdrup 2–3 km and eventually rising back up to the surface in the Southern Ocean. Antarctic bottom water (AABW) is formed Significance around Antarctica and spreads northward into the abyssal basins. EARTH, ATMOSPHERIC, The AABW that makes it into the Atlantic then slowly upwells AND PLANETARY SCIENCES into the lower NADW before returning southward and resur- To understand climatic swings between glacial and interglacial facing again around Antarctica (1, 2). The glacial equivalent climates we need to explain the observed fluctuations in of NADW was likely confined to shallower depths, leaving atmospheric carbon dioxide (CO2), which in turn are most more of the deep Atlantic filled with water masses originat- likely driven by changes in the deep ocean circulation. This ing primarily from around Antarctica (3–5). Moreover, the two study presents a model for differences in the deep ocean water masses appear more distinct, with less mixing between circulation between glacial and interglacial climates consis- them (6). tent with both our physical understanding and various proxy Multiple studies have pointed toward the potential impor- observations. The results suggest that observed changes in tance of sea ice and surface buoyancy fluxes around Antarc- ocean circulation and stratification are caused directly by tica in controlling changes in the deep ocean stratification and atmospheric cooling or warming, which has important impli- circulation between the present and Last Glacial Maximum cations for our interpretation of glacial–interglacial transi- (LGM) (7–11). Specifically, we recently showed that enhanced tions. In particular, the direct link between atmospheric tem- buoyancy loss around Antarctica is expected to lead to an perature and ocean circulation changes supports the notion of increase in the abyssal stratification, an upward shift of NADW, a positive feedback loop between atmospheric temperature, and a clearer separation between NADW and southward-flowing ocean circulation, and atmospheric CO2. AABW (11)—all in agreement with inferences made for differ- ences in circulation and stratification between the present and Author contributions: M.F.J. designed research, performed research, analyzed data, and LGM (3, 6, 12, 13). This gives rise to the hypothesis that strong wrote the paper. cooling around Antarctica led to an increased net freezing rate. The author declares no conflict of interest. The resulting net salt flux into the ocean amounts to an increased This article is a PNAS Direct Submission. buoyancy loss, which then led to the observed changes in deep 1Email: [email protected]. ocean stratification and circulation. This hypothesis is tested in This article contains supporting information online at www.pnas.org/lookup/suppl/doi:10. this study. 1073/pnas.1610438113/-/DCSupplemental. www.pnas.org/cgi/doi/10.1073/pnas.1610438113 PNAS j January 3, 2017 j vol. 114 j no. 1 j 45–50 Downloaded by guest on September 23, 2021 proxy data for the LGM, the prescribed atmospheric cooling is polar amplified, ranging from 2◦C in the tropics to 6◦C around Antarctica (14, 15). All other boundary conditions are held fixed. The reduction in atmospheric temperature leads to a reduction in ocean surface temperature and an expansion of sea ice around Antarctica, as well as the appearance of sea ice in the North Atlantic (Fig. 1B). Moreover, the model suggests a cooling and salinification of AABW, leading to a strong salt stratification in the deep ocean, which replaces the reversed salinity stratifica- tion observed with present-day forcing (Fig. 2B). The strong salt stratification is consistent with pore-fluid data for the LGM (12). (To compare the absolute salinity values here to those inferred for the LGM, one needs to account for the increased bulk ocean salinity resulting from the eustatic drop in sea level, which is not included in the simulations and would add around 1 g/kg glob- ally.) The AMOC cell weakens and shoals (Fig. 3B), again consis- tent with inferences for the LGM (3–5). The abyssal cell slightly strengthens and becomes somewhat more confined to the abyss, leading to an increased separation between the two overturning cells, which may have played an important role in the increased ocean carbon storage (6). Fig. 1. (A and B) Sea surface temperature (colors) and sea-ice concentration In the real ocean the abyssal overturning cell is distributed (white contours), for simulations with boundary conditions representing over multiple basins and currently overlaps in depth and density present-day conditions (A) and LGM conditions with reduced atmospheric with the AMOC cell, leading to a continuous exchange of water temperature (B). Contour interval for sea-ice concentration is 20%. between the two cells in the Southern Ocean (1, 2). Whereas this interbasin overlap between the present-day overturning cells cannot be modeled in a single-basin model, the simulated con- (SV) and −5.2 SV are roughly consistent with the observed rate traction of both the AMOC and abyssal cells is likely to be of NADW formation and the transport of AABW into the North robust (11). In a multibasin configuration, this contraction of Atlantic (1, 2). both cells is expected to remove or reduce the overlap between We now analyze the response of the model’s equilibrium solu- the two cells, thus generating a more isolated abyssal water mass. tion to a reduction in atmospheric temperature. Consistent with The rearrangement of the overturning circulation would likely Fig. 2. (A–D) Zonal mean temperature (A and B) and salinity (C and D) for simulations with boundary conditions representing present-day forcing (A and C) and simulations with reduced atmospheric temperature resembling LGM conditions (B and D). Contour intervals are 1◦C in A and B and 0:05 g/kg in C and D. Note that the colorbar ranges have been cropped to focus on the deep ocean. 46 j www.pnas.org/cgi/doi/10.1073/pnas.1610438113 Jansen Downloaded by guest on September 23, 2021 Fig. 3. (A and B) Meridional overturning streamfunction (colors) and potential density referenced to 2 km depth (black lines), for simulations with boundary conditions representing present-day forcing (A) and LGM conditions with reduced atmospheric temperature (B). The overturning streamfunction is computed from the sum of the Eulerian zonal mean velocity and the parameterized eddy-induced bolus velocity.
Details
-
File Typepdf
-
Upload Time-
-
Content LanguagesEnglish
-
Upload UserAnonymous/Not logged-in
-
File Pages6 Page
-
File Size-