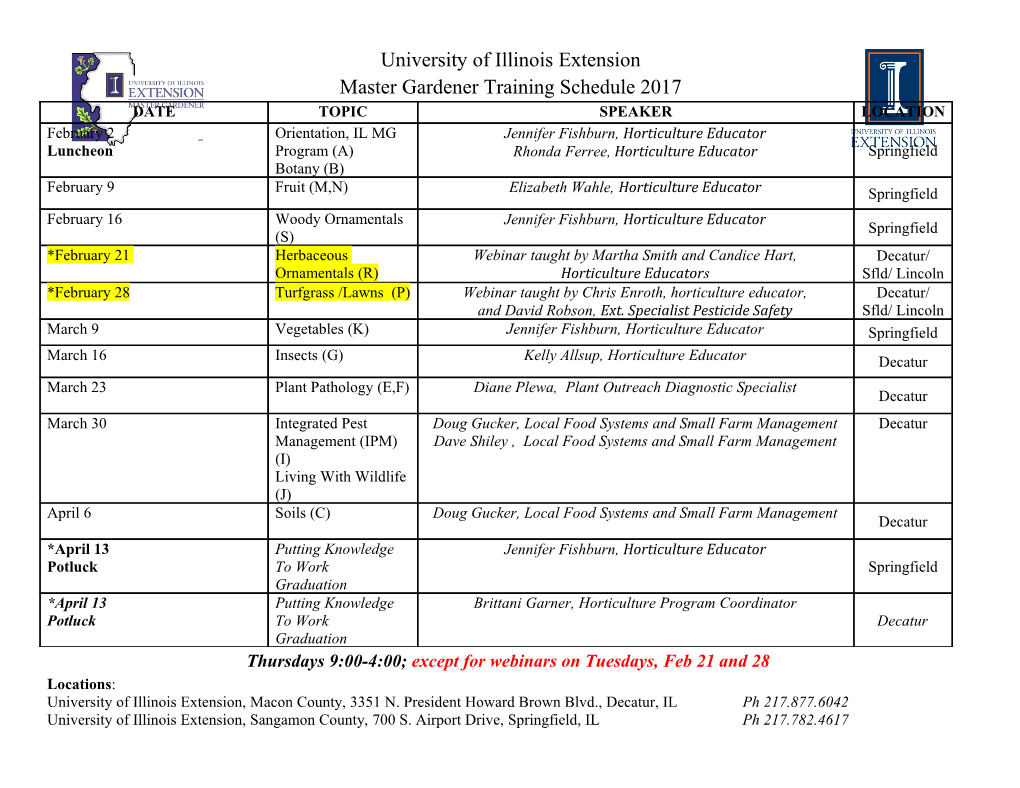
P/381 USA Plasma Diagnostic Developments in the UCRL Pyrotron Program By C. B. Wharton,* J. C. Howard* and O. Heinzf conditions of the plasma are determinable and if the At the outset of the Berkeley and Livermore pro- 4 5 grammes in the study of dense, high temperature, electron energy distribution is known ' a meaningful transient plasmas of large physical extent, it was value for electron kinetic temperature may be cal- apparent that the existing diagnostic techniques culated. could not yield a complete picture of physical processes Since a plasma is highly dispersive in the micro- in the plasma.1 High temperature extensions of wave part of the spectrum the chosen frequency is diagnostic theories were not understood. Few theories determined by the plasma density and size. The had been substantiated experimentally. Worse yet, diversity of experiments undertaken in the Pyrotron some of the instruments which had become standards programme has necessitated utilizing microwave for plasma research (such as Langmuir probes and equipment in the following frequency bands: 8-12 kMc, 19-26 kMc, 32-48 kMc and 68-77 kMc (kMc = probing electron beams) introduced such serious per- 9 turbations by their presence that the validity of the kilomegacycles—10 cycles per second). Very recently results was questionable. some experiments at 130-150 kMc have been contem- This paper describes techniques developed in con- plated. In order to determine density and collision nection with the Pyrotron programme to fill in some frequency, the plasma must be transparent. In order of these voids. to obtain sufficiently intense radiation from the plasma to be measurable the region should be nearly opaque. SURVEY OF DIAGNOSTIC METHODS Therefore, in order to make both measurements During the course of the various experiments simultaneously, they must be performed at different numerous diagnostic approaches were tried. It would frequencies. require considerably more space than the scope of this The pyrotron configuration permits a variety of paper permits to present explicit discussions of more modes of coupling into the discharge. Transmission than a representative group of these techniques. across the discharge region with the rf electric field However, for completeness, a brief tabulation of either parallel or perpendicular to the machine essentially all of the important techniques tried is magnetic field allows coupling with or decoupling presented in Tables 1, 2 and 3. A more complete dis- from the electron cyclotron interactions. Propagation cussion of the most commonly used methods is along the magnetic lines with circularly-polarized presented in the remaining sections of this paper and waves allows coupling to either the œ — шс or o> + coc in a group of papers, P/377-380, of the preceding modes. The inhomogeniety of the magnetic field is session, A-9. usually a source of ambiguity but in some cases it permits the position of the plasma boundary to be Microwave Interactions determined by observing the location at which Diagnostic techniques involving microwave inter- gyroresonance occurs. actions with plasmas2»3 have been in use at this Figure 1 shows a sketch of a typical microwave laboratory since 1952. Our approach has been to diagnostics system. To measure absorption, the measure the phase shift, the absorption and the 60-foot reference path is removed2 and the klystron scattering of a wave propagating through the plasma is modulated by random noise in order to average out region, determine the plasma spatial distribution by a any internal reflections within the discharge chamber. second method (light emission, probes, etc.) and The noise spectrum is some 200 Me wide. calculate the electron density and collision frequency. The radiation receiver is a wide-band (10 Me) Simultaneously the "white" noise radiation from the receiver with a threshold sensitivity of about 0.1 plasma is monitored by a calibrated microwave super- micro-microwatt. Detected noise is viewed directly, heterodyne receiver. If the opacity or geometrical without time averaging, to preserve the wide band- * University of California Radiation Laboratory, Livermore, width. California. In order to seek correlation between the microwave f Stanford Research Institute, Menlo Park, California. and other measurements, a control experiment has 388 PLASMA DIAGNOSTIC DEVELOPMENTS 389 been devised. Plasma is generated within a stable radiation appears only when the plasma becomes PIG (Philips Ion Gauge) configuration by passing opaque,2 even though the electron temperature re- pulses of 4-8 msec duration, 0.1-2 amp magnitude, mains high during a large fraction of the pulse. 60 times per second through a gas (He, Щ, A, etc.) The opacity dependence of the radiation has been at pressures ranging between 0.1 and 20 ¡i Hg. Electron demonstrated also in the high compression pyrotrons. densities as high as 1013 per cm3 are easily obtained, The right-hand circularly polarized wave suffers large and electron kinetic temperatures as high as 25 ev absorption by a plasma at a frequency slightly below have been measured. Figure 2 shows a sketch of the gyrofrequency, even when the density and collision equipment and Fig. 3 shows a composite oscilloscope frequency are relatively low. The absorption (and display of data. It is apparent that the microwave radiation, by Kirchhoff's law) cross sections due to Table 1. Plasma Ion Diagnostics Plasma diagnostic Employed Experimental application Ъпа comments Performance technique on* valuation^ Ion energy- t-t Magnetic momentum analysis, followed by electro- B-l analyzer static energy analysis. Min. ion current 1 ma; ion energy range 16 ev to 20 kev. Yields distributions only if conditions are reproducible from discharge to discharge. Time-of-flight Q-c Velocity measurement of plasma burst from pulsed A-l measurement Fel. source using probe or microwave sampling. Calorimetric P-4 Measures temperature increase of target when im- D measurement T-T mersed in plasma. Employs thermocouples, optical instruments, or rate of deterioration of target. Fel. Determination of plasma position and ion orbit sizes. Fluorescent ALB. Qualitative density measurement. Time-resolved or screen t-t integrated presentation. T-T Charged All Faraday cups, biased and unbiased to determine particle particle escape rates. Single and double probes to collectors determine ion and electron densities. Neutral Fel. Determines high energy neutral particle flux by B-2 particle measurement of secondary electron current. Used for detector determination of charge exchange loss rate. Optical All Doppler shift and broadening measured by spectro- B-2 spectrum but graph or interferometer. Determines random and analysis Fel. collective ion velocities. Stark broadening to deter- mine ion density. Spectral line identification. a Key to apparatus code: b Key to evaluation code: ALB ALBEDO: DC mirrors, ultra high vacuum (no longer active). A Highly reliable. Fel. FELIX: High energy injection, ultra high vacuum. В Moderately reliable. Gup. GUPPY: PIG in DC mirrors. С Poor reproducibility. L-P LITTLE PIG: Diagnostics correlation. D Not sufficiently evaluated P-4 Plasma source experiment. 1 Quantitative results. Q-C DC Variable mirror ratio experiment (no longer active). 2 Semi-quantitative. Sat. SATURN: High compression, radial Injection. 3 Qualitative. t-t TOY TOP: High compression, multiple stage. T-T TABLE TOP: High compression, collision injection. this "cyclotron'' interaction are so large that the electrons reach radiative equilibrium rapidly.4»5 Optical Observations Thus, the thermalizing time for the electrons to assume Spectroscopic measurements have been made, a Maxwellian energy distribution is many orders of wherever possible, in connection with the pyrotron magnitude shorter than that for the ions. In the experiments. The general procedure has been to theories for the microwave transmission and radiation identify first the light emitters in a particular plasma measurements performed on pyrotrons, the assump- by means of photographic spectra covering as wide a tion of a Maxwellian electron distribution, then, seems frequency range as is feasible. Next, the time depen- justified, and the "temperatures" measured have dence of atomic or molecular features to which some meaning. Figures 4 and 5 illustrate the opacity inter- particular interest is attached, e.g. those of D, dependences. The signal amplitudes correspond to He+, C+++, Ti+, C2, CH, etc., is obtained. An attempt electron temperatures of 15 to 25 kev. The electron is then made to learn something about the conditions density before compression was ordinarily between in the plasma from spectral Une intensity ratios or 1012 and 1013/cm3. The collision frequency never the intensity distribution within a given Une. Often exceeded 107 per second. the thermal Doppler broadening in the case of the 390 SESSION A-10 P/381 С. В. WHARTON et al. BALANCED IN26 the high electron kinetic temperatures (1-6 kev) MIXER ф achieved in the Saturn experiment, the high light output during initial ionization makes possible some interesting optical measurements. A determination of DUAL BEAM SCOPE ion density by observation of Stark broadening of the CHAN В Ha line was accomplished by utilizing a Wratten No. 25A filter, as a monochromator, and a Fabry- Perot interferometer. The fringes were observed with a camera directly and also through an image converter to permit time resolution of the fringe broadening. 6254 К — BAÑO Kodak No. IF-3 photographic plates provided KLYSTRON sufficient sensitivity and yet small enough grain size to facilitate microscopic scanning. Since the optical POWEt R SUPPLY system is all transparent, the camera is able also to 381.1 observe the interior of the discharge region to record Figure 1. Microwave diagnostics system. Fringe-shift interfero- the location from which radiation emanates. Figure 6 meter and plasma radiation receiver illustrates the experiment geometry and Fig. 7 shows the broadened fringes with the discharge chamber ELECTROMAGNET visible through the interferometer. Apparently the light comes from a ball at the center of the chamber. A composite data analysis is shown in Fig.
Details
-
File Typepdf
-
Upload Time-
-
Content LanguagesEnglish
-
Upload UserAnonymous/Not logged-in
-
File Pages6 Page
-
File Size-