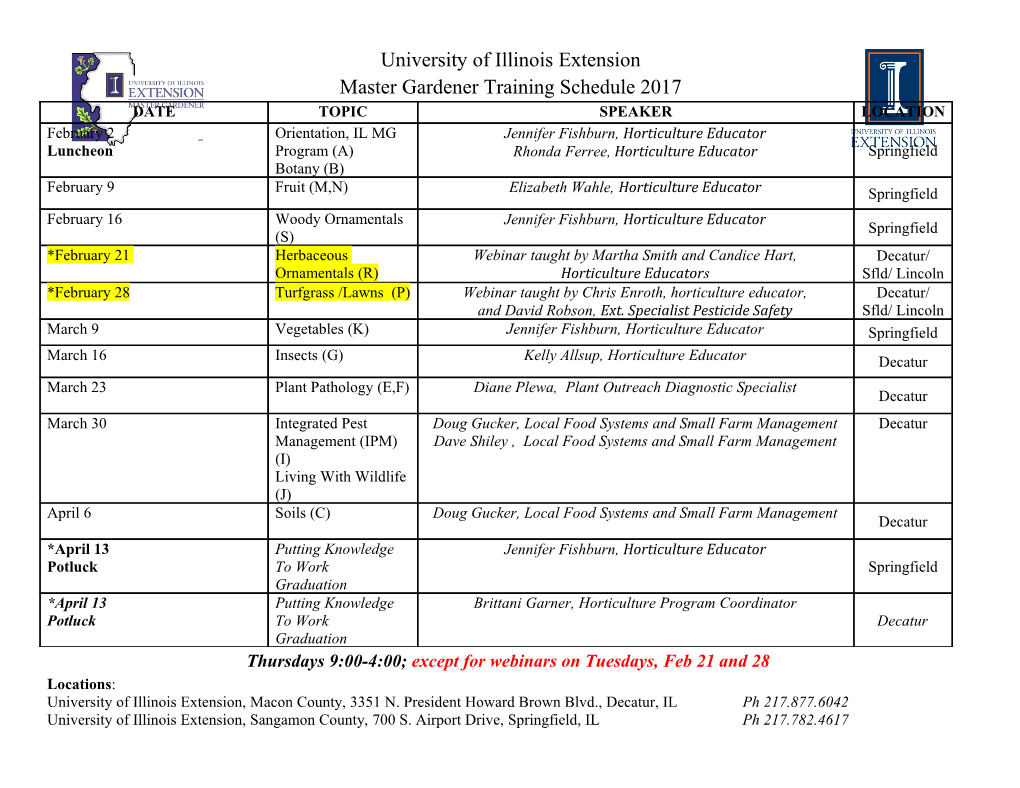
The crystal chemistry, dissolution kinetics and dehydroxylation of oxide-type lateritic Ni ore Matthew Landers BSc (Earth Science), Honours This thesis is presented for the degree of Doctor of Philosophy at the University of Western Australia School of Earth and Environment University of Western Australia 2010 TABLE OF CONTENTS Page Number ABSTRACT………………………………………………………………... I ACKNOWLEDGMENTS…………………………………………………. III PUBLICATIONS ARISING FROM THIS THESIS………………………. IV STATEMENT OF CONTRIBUTION……………………………………... IV Chapter 1: INTRODUCTION…………………………………………… 1. 1.1. Introduction……………………………………………………………. 1. 1.2. References……………………………………………………………... 16. Chapter 2: RAPID DEHYDROXYLATION OF NICKELIFEROUS GOETHITE IN LATERITIC NICKEL ORE: X-RAY DIFFRACTION AND TEM INVESTIGATIONS …………………….. 21. 2.1. Abstract………………………………………………………………...22. 2.2. Introduction………………………………………………………......... 23. 2.3. Materials and Methods…………………………..……………………..25. 2.4. Results and Discussion………………………………………………....28. 2.2.1. Mineralogy/ chemistry of goethites…………………………28. 2.2.2. Dehydroxylation temperature and weight loss due to heating………………………………………………. 29. 2.2.3. Unit cell dimensions……………………………………….. 39. 2.2.4. XRD line broadening/ Mean Coherent Length (MCL)……..42. 2.2.5. Surface Area………………………………………………...45. 2.2.6. Crystal morphology…………………………………………46. 2.5. Conclusions…………………..………………………………………...53. 2.6. Acknowledgments……………......…………………………………….53. 2.7. References…………………………..………………………………….54. Chapter 3: DISSOLUTION KINETICS OF DEHYDROXYLATED NICKELIFEROUS GOETHITE FROM LIMONITIC NICKEL ORE………………………………………………………………………... 57. 3.1. Abstract………………………………………………………………... 58. 3.2. Introduction……………………………………………………………. 58. 3.3. Materials and Methods………………………………………… ……... 60. 3.3.1. Samples……………………………………………………… 60. 3.3.2. Heating procedure…………………………………………… 61. 3.3.3. Surface area………………………………………………….. 61. 3.3.4. X-ray diffraction analysis…………………………… ………61. 3.3.5. Acid dissolution experiment………………………………… 62. 3.3.6. Transmission electron microscopy………………………….. 62. 3.4. Results and Discussion………………………………………………... 62. 3.5. Conclusions……………………………………………………………. 78. 3.6. Acknowledgments……………………………………………………...79. 3.7 References ……………………………………………………………... 79. Chapter 4: NICKEL, AL AND CR DISTRIBUTION AND SPECIATION IN RAPIDLY DEHYDROXYLATED GOETHITE IN OXIDE-TYPE LATERITIC NICKEL ORES………………………. 82. 4.1. Abstract………………………………………………………………... 83. 4.2. Introduction……………………………………………………………. 83. 4.3. Materials and Methods………………………………………… ………85. 4.4. Results and Discussion………………….…………………………….. 88. 4.4.1. Mineralogy and chemistry…………………………………... 88. 4.4.2. Metal distribution in goethite crystals………………………..92. 4.4.2.1. Congruency of acid dissolution…………………… 92. 4.4.2.2. EFTEM mapping………………………………….. 98. 4.4.3. Mineralogical changes due to heating………………………..99. 4.4.4. Metal distribution in dehydroxylated goethite: OH- hematite and hematite……………………………………… ………102. 4.5. Conclusions……………………………………………. ………………111. 4.6. Acknowledgments……………………………………………………...112. 4.7. References……………………………………………………………... 112. Chapter 5: NICKEL DISTRIBUTION AND SPECIATION IN RAPIDLY DEHYDROXYLATED GOETHITE IN OXIDE-TYPE LATERITIC NICKEL ORES: X-RAY ABSORPTION SPECTROSCOPIC INVESTIGATION………………………………… 117. 5.1. Abstract………………………………………………………………... 118. 5.2. Introduction……………………………………………………………. 118. 5.3. Materials and Methods………………………………………………… 121. 5.3.1. Samples……………………………………………… 121. 5.3.2. XAFS Data collection……………………………….. 121. 5.3.3. XAFS Data analysis…………………………………. 122. 5.4. Results and Discussion………………………………………………... 123. 5.4.1. XANES……………………………………………………… 123. 5.4.1.1. A) Pre-edge peak; 1s to 3d electron transitions…… 125. 5.4.1.2. B) 1s to 4pz transition and shakedown electron Transition…………………………………………………... 126. 5.4.1.3. Ci and Cii) 1s to 4p electron transfer: the whiteline.. 127. 5.4.1.4. D) Beyond the main-edge jump…………………… 128. 5.4.2. EXAFS………………………………………………………. 128. 5.4.2.1. Lateritic Ni-goethite……………………………….. 128. 5.4.2.2. Nickeliferous goethite heated at 340/400°C (OH-hematite) and 800°C (hematite)……………………….134. 5.5. Conclusions……………………………………………………………. 138. 5.6. Acknowledgments……………………………………………………...139. 5.7. References……………………………………………………………... 139. Chapter 6: REVIEW DISCUSSION/ SUMMARY……………………...142. 6.1. Mineralogy and geochemistry…………………………………………. 142. 6.2. Location and distribution of Ni, Al and Cr in goethite in oxide-type lateritic Ni ore………………………………………………………….142. 6.3. Dissolution kinetics of lateritic goethite………………………………. 144. 6.4. Rapid dehydroxylation of goethite to hematite………………………... 145. 6.4.1. Dehydroxylation of goethite to hematite: Mineralogy/ Morphology…………………………………………………………145. 6.4.2. Dehydroxylation of goethite to hematite: Effect on dissolution rate……………………………………………………... 147. 6.4.3. Rapid dehydroxylation of goethite to hematite: Effects on location and distribution of Ni, Al and Cr…………………………..147. 6.5. Relevance to the mining industry……………………………………... 149. 6.6. Where to from here?................................................................................150. 6.7 References ………………………………………………………………151. I ABSTRACT The recent strong demand for Ni for steel production has significantly depleted the easily extractable Ni sulphide deposits, causing the mining industry to look towards the more abundant albeit complex, low-grade lateritic Ni deposits. There have been major difficulties in the extraction of Ni from lateritic Ni ores reflecting, inter alia, the limited knowledge of the location and distribution of Ni within minerals (particularly iron- oxides) in lateritic ores, as well as the limited understanding of the acid leaching kinetics of these minerals. A new method for improving the extraction of Ni from oxide-type (limonitic) lateritic Ni ores by means of shock heating has been investigated in this thesis utilising a combination of bulk and macroscopic techniques. Five oxide-type lateritic Ni ores from Indonesia (Weda Bay), Western Australia (Kalgoorlie and Ravensthorpe) and New Caledonia (Goro and Koiambo), composed mostly of acicular, nano-sized goethite crystals with minor amounts of quartz, talc, willemseite, maghemite, magnetite, asbolane, kaolinite, Mn oxides and various spinels. These minerals were identified using synchrotron X-ray diffraction (SXRD) and electron energy loss spectroscopy (EELS). The nickeliferous goethites were subjected to shock heating for 30 minutes at temperatures in the range 220-800°C. Goethite partially dehydroxylated to OH-hematite at 300-400°C and had completely altered to well ordered hematite at 800°C. OH-hematite has broad XRD reflections, structural water, high specific surface area, structural disorder and small crystal size. The high surface area (1.5-2.6 fold increase from that of goethite) is due to the formation of slit-shaped micropores (300°C), which further develop into elliptically-shaped micropores (400°C), and is partly responsible for a 9-34 fold increase in the dissolution rate constant k for the Kabai equation, measured from the dissolution of Fe in 2M H2SO4. The remaining 5-10 fold increase in the dissolution rate constant k is presumably due to the higher density of structural defects in OH-hematite. The presence of structural Al and Cr in goethite was shown to reduce dissolution rate possibly through the greater Me-OH, O bond strength relative to Fe, Ni-OH, O. Rapid high temperature dehydroxylation (800°C) of goethite resulted in the formation of well ordered hematite with no structural water, low surface area and with high structural order. The low surface area is due to the removal of most micropores and the development of extensive ordered hematite domains by surface and volume diffusion. II This results in a significant decrease (~1.5-2.5 fold) in the dissolution rate constant k for the Kabai equation relative to values for OH-hematite. For most of the samples the Ni K-edge extended X-ray absorption fine structure (EXAFS) spectroscopy results showed interatomic distances indicative of Ni substituting for Fe in goethite. Rapid dehydroxylation of goethite to form OH-hematite and hematite significantly affected the distribution of Ni in the iron oxides. The acid dissolution for Fe versus Ni was incongruent and indicative of rapid dissolution of surface located Ni, or formation of a discrete Ni mineral. Corresponding Ni K-edge EXAFS and energy filtered transmission electron microscopy (EFTEM) results for 800°C heated samples (i.e. hematite) showed evidence of some Ni migrating to the surface of the neoformed hematite. The Ni K-edge EXAFS results showed four distinct metal neighbours representing Fe/Ni in face-sharing (RNi-Fe~2.88-2.92 Å) and three different corner-sharing complexes (RNi-Fe~3.37- 3.41 Å, RNi-Fe~3.62-3.64 Å and RNi- Fe~3.92-4.08 Å). The first three interatomic distances represent Ni substituting for Fe in hematite, while the fourth is indicative of an inner sphere surface complex. There was no evidence for the formation of a discrete Ni mineral, suggesting most of the Ni is retained in the structures of the neoformed hematite, although some Ni migrates to the surface of the hematite where it forms a surface complex. These results may contribute to the development of more efficient extraction methods for Ni in oxide-type lateritic Ni ores. The rate of dissolution of goethite in acid in heap and pressure leach facilities will be enhanced by shock heating at 340-400°C where microporosity and structural disorder is maximised. Similarly, shock heating nickeliferous goethite to form well ordered hematite at 800°C,
Details
-
File Typepdf
-
Upload Time-
-
Content LanguagesEnglish
-
Upload UserAnonymous/Not logged-in
-
File Pages161 Page
-
File Size-