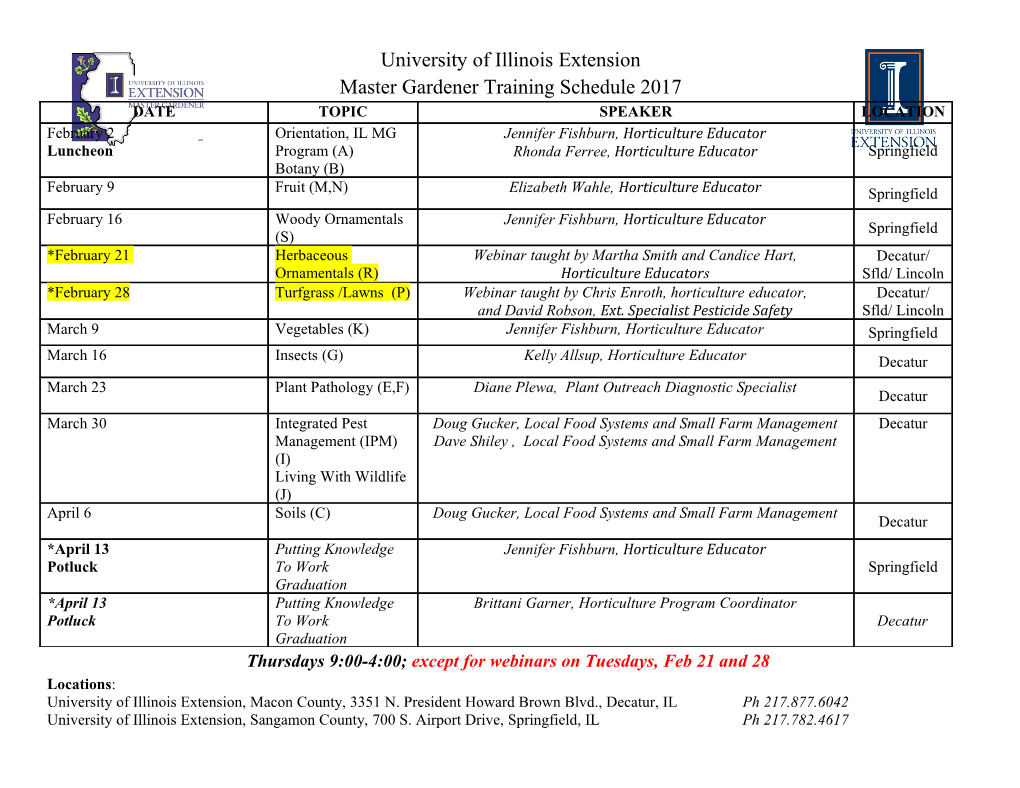
A novel class of winged helix-turn-helix protein: the DNA-binding domain of Mu transposase Robert T Clubb1 , James G Omichinski1 , Harri Savilahti2 , Kiyoshi Mizuuchi 2, Angela M Gronenborn1 * and G Marius Clore1* 1Laboratory of Chemical Physics and 2 Laboratory of Molecular Biology, Building 5, National Institute of Diabetes and Digestive and Kidney Diseases, National Institutes of Health, Bethesda, MD 20892-0520, USA Background: Mu transposase (MuA) is a multidomain seven-residue turn connecting them comprise a helix- protein encoded by the bacteriophage Mu genome. It turn-helix (HTH) motif. In addition, there is a long is responsible for translocation of the Mu genome, nine-residue flexible loop or wing connecting strands B2 76 which is the largest and most efficient transposon and B3 of the sheet. NMR studies of MuA complexed known. While the various domains of MuA have been with a consensus DNA site from the internal activation delineated by means of biochemical methods, no data region of the Mu genome indicate that the wing and have been obtained to date relating to its tertiary the second helix of the HTH motif are significantly structure. perturbed upon DNA binding. Results: We have solved the three-dimensional solution Conclusions: While the general appearance of the 76 structure of the DNA-binding domain (residues 1-76; DNA-binding domain of MuA is similar to that of MuA7 6) of MuA by multidimensional heteronuclear other winged HTH proteins, the connectivity of the NMR spectroscopy. The structure consists of a three- secondary structure elements is permuted. Hence, the membered -helical bundle buttressed by a three- fold of MuA 76 represents a novel class of winged HTH stranded antiparallel P-sheet. Helices HI and H2 and the DNA-binding domain. Structure 15 November 1994, 2:1041-1048 Key words: DNA-binding domain, Mu transposase, NMR spectroscopy, winged helix-turn-helix motif Introduction which bind an internal activation sequence and the ends Transposons are mobile genetic elements capable of of the phage genome, respectively [6,7]. In this paper we translocation from one site on the DNA to another. present the first structure determination of any domain Transposition, the recombination reaction utilized to related to the Mu transposase/integrase family of accomplish this task, is also the mechanism employed to proteins, namely the solution structure of the amino- integrate cDNA copies of retroviral and retrotransposon terminal DNA-binding domain (MuA76 , residues 1-76) RNA into the chromosomal DNA of their host. of the Mu transposase by means of multidimensional Typically, transposition involves a single protein which heteronuclear NMR spectroscopy. MuA76 possesses a performs two chemical steps: cleavage of the ends of the novel topology and represents a new class of winged transposon DNA, followed by strand transfer which leads helix-turn-helix (HTH) DNA-binding domain. In to the covalent linkage of the donor DNA ends to its addition, we show that the flexible loop comprising the target host DNA site. The largest and most efficient wing and the second helix of the HTH motif are transposon known is the bacteriophage Mu genome, significantly perturbed upon binding to a consensus which uses the phage-encoded transposase (MuA DNA site derived from the internal activation sequence protein) to pair the ends of the phage DNA, cleave the of the Mu genome. termini, and promote strand transfer [1,2]. Mu transposase is a monomeric 75 kDa protein which Results functions as a tetramer during transposition. Assembly Structure determination into the active tetramer requires multiple sequence The solution structure determination was carried out elements on the donor DNA, including sites located at using 1 5N and 15N/ 13 C labeled samples and involved the the ends of the genome and an enhancer-like element application of double and triple resonance three-dimen- within the Mu genome [3-5]. Mu transposase can be sional (3D) and 4D NMR spectroscopy [8-10]. divided into three structurally distinct domains, each Examples of the quality of the NMR data are provided with specific functions. The amino-terminal domain by planes of the 4D 15 N/ 13 C and 13C/ 13C-edited (30 kDa) is responsible for sequence-specific DNA nuclear Overhauser enhancement (NOE) spectra shown binding and can further be subdivided into two separate in Fig. 1. An iterative strategy was employed for subdomains, comprising residues 1-76 and 77-247 the structure determination [11,12]. The final structure *Corresponding authors. Current Biology Ltd ISSN 0969-2126 1041 1042 Structure 1994, Vol 2 No 11 calculations were based on 1192 approximate interproton solution. The remainder of the structure (residues 3-36 distance restraints derived from the 3D and 4D hetero- and 45-65) is well defined with a precision of 0.36+0.06 nuclear-edited NOE spectra, supplemented by 18 A for the backbone atoms, 0.74+0.05 A for all atoms distance restraints for 9 backbone hydrogen bonds and 0.36±0.05 A for all atoms that do not exhibit (which were only introduced in the final stages of the conformational disorder. refinement), 74 torsion angle restraints (40 , 23 X1 and 11 X2), 36 3 JHNa coupling constant restraints, and stereo- Description of the structure specific assignments for 17 of the 49 [5-methylene The structure of MuA7 6 comprises a three-helical bundle groups, and for the methyl groups of four of the five buttressed by a three-stranded antiparallel -sheet with valine residues and five of the nine leucine residues. an overall B1-H1-T-H2-B2-W-B3-H3 topology (where Stereospecific assignments, and 04 and X1 torsion angle B, H, T and W stand for 3-strand, a-helix, turn and restraints were obtained by means of a conformational wing, respectively) (Figs 2a and 3). The overall fold of grid search on the basis of 3JHN and 3 j coupling the protein is asymmetric and consists of a globular constants and intra-residue and sequential inter-residue protein core and a protruding loop. Strand B1 NOEs [13]. Information from 3JHN[ and 3JCOHP was (Trp4-Ser6) leads into the short helix H1 (Pro7-Alall). also employed for identifying the appropriate Xl rotamer A long seven-residue turn from Asnl2 to Lysl8 connects and for detecting rotamer averaging, and in addition, helix H1 with helix H2 (Thr19-Gln30). The arrange- 3 3 JCYN and JcYco coupling constants were used for the ment of the H1-T-H2 segment is similar to the HTH determination of the side chain conformation of DNA-binding motif used by a variety of proteins, threonine and valine residues. The 11 X2 torsion angle although the turn is significantly longer than the usual restraints comprised restraints for five leucine residues four to five residues [17,18]. Strand B2 (Trp32-Arg35) (Leu3, LeulO, Leul3, Leul6, Leu53 and Leu62), Ile24, follows the HTH motif and pairs with strand B3 and the aromatic rings of Tyr25, Tyr48, Trp4 and Trp32. (Ala45-Asn49) which in turn pairs with strand B1. A The X2 torsion angles for leucine and isoleucine residues large partially disordered loop extending from Thr36 to were obtained from 3 JCC coupling constants and the Lys44, which we refer to as a wing, connects strands B2 pattern of intra-residue NOEs [14]. The X2 angles of the and B3 and protrudes from the globular core. The two tyrosine residues were restrained to 90+30 ° , and the remainder of the protein consists of strand B3 and the ° X2 angles of Trp4 and Trp32 were restrained to 90+30 carboxy-terminal helix H3 (Val55-Gln65) which pack and -90±30 ° , respectively [15,16]. The latter two against strand B1 and helix H1 to close off the protein restraints were only introduced in the final stages of the core. The hydrophobic core of the protein comprises refinement. The structural statistics and atomic root Leu3 and Val5 of strand B1; LeulO of helix H1; Leul3 mean square (rms) differences for the final ensemble of and Leul6 of the turn between helices H1 and H2; 34 simulated annealing structures, based on a total of Va123, Ile24, Ala27 of helix H2; Trp32 of strand B2; 1320 experimental NMR restraints, are summarized in Tyr48 of strand B3; Leu53 of the turn between strand B3 Tables 1 and 2, respectively, and a stereoview of a best fit and helix H3; and Leu61 of helix H3 (Fig. 2b). superposition of the backbone atoms and ordered side chains is shown in Fig. 2. The amino terminus (residues Interaction with DNA 1 and 2), carboxyl terminus (residues 66-76) and a To identify the location of the DNA-binding site on protruding loop (residues 37-44) are disordered in MuA 76 we carried out NMR studies on a complex 3 (b) 4Di13 C!3 C-NOESY (a)4D15N1 C-NOESY 15 1 13 N12 HN N F3 = 114.25, H F4= 8.75 L16 61 C F3= 23.88,1H F4= 1.13 : L1651L i -, V26y1 V23y2 C Ali1, 1.0 1.0 L162-4 p L16y-, A571 2.0 2.0 co I L1 6's O 3.0-" N12P'S. 0 3.0 a 3 30 3 1 e P1762 4.0 Fig. 1. Example of 1 C(F )- H(F ) planes 15 131 2 P Alla of (a) the 4D N/ C-edited NOE 4.0 spectrum and (b)the 4D 13C'3C-edited K18xa 76 O N12a 5.0 NOE spectrum of MuA . The destina- 0 L16a _ tion proton in (a) is the NH group of _ Asnl2 and in (b) is the C8 H3 methyl 55.0 50.0 45.0 40.0 73.0 68.0 63.0 group of Leu16.
Details
-
File Typepdf
-
Upload Time-
-
Content LanguagesEnglish
-
Upload UserAnonymous/Not logged-in
-
File Pages8 Page
-
File Size-