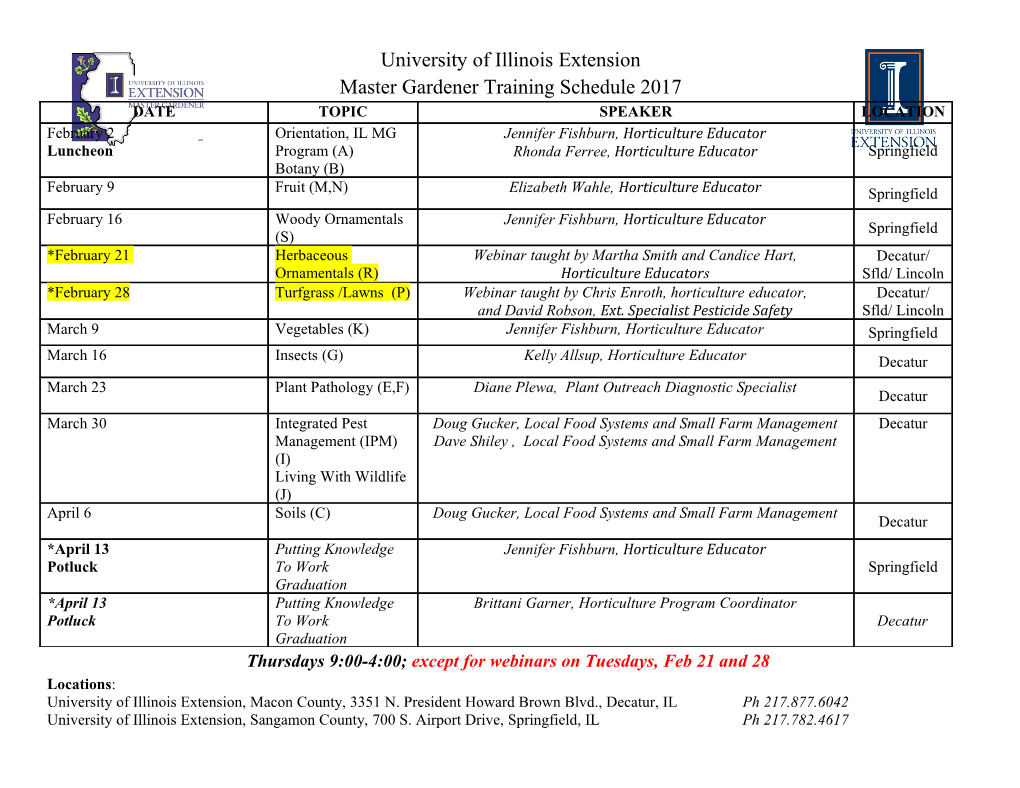
BULLETIN OF MARINE SCIENCE, 39(2): 145-161, 1986 LARVAL INVERTEBRATE WORKSHOP INTRODUCTION TO THE INVERTEBRATE LARVAL BIOLOGY WORKSHOP: A BRIEF BACKGROUND R. Andrew Cameron The larvae of benthic marine invertebrates are puzzling creatures. They are usually minute, yet they are anatomically complex. They remain obscure among the more numerous holoplanktonic animals with whom they live, yet they play a visibly important role in benthic population dynamics. Because small and obscure larvae seldom reveal either their origins, destinations or hour-by-hour activities, they represent the life phase that is least understood in invertebrate life patterns. To replace missing data, overly simple assumptions have been used in models of community ecology and population biology. Lack of information on larval dispersal, larval nutrition and predation and the role of larvae in life history strategies is particularly apparent. Productive research into larval lives and processes continues to accumulate and for our purposes here can be divided into four areas: (1) dispersal, (2) settlement and metamorphosis, (3) life history ecology and (4) evolution. Dispersal That the larval stage is the dispersal phase of benthic marine invertebrate life cycles is undeniable (see Scheltema this volume for review). However, only a few of the larger decapod larvae can swim faster than ocean currents and thus take an active role in their horizontal distribution (Mileikovsky, 1973). Larvae have been characterized as passively transported particles at the mercy of the currents in which they find themselves. In the Kiel Bight, the larvae of most polychaetes and echinoderms do not make significant vertical migrations, nor do they pass the discontinuity layers (Banse, 1955; 1956). Hydrographic mechanisms alone can explain the greater abundance of larvae around tropical islands or reefs and within atoll lagoons (Boden, 1952; Johnson, 1954; Hamner and Hauri, 1982). Horizontal discontinuities such as surface slicks, generated by internal waves (Fu and Holt, 1982), contain higher abundances of larvae and other plankton (Zeldis and Jillett, 1982; Shanks, 1983). But larvae unable to swim against currents can still control their movement by changing vertical position in a water column with currents which move in different directions. This principle is best illustrated by the retention of larvae in estuaries which possess a net seaward flow. The retention of larvae in estuaries may be a passive phenomenon akin to the retention of fine sediments (Korringa, 1952; DeWolff, 1974), but it is more likely that biological adaptations increase the probability of retention. For example, the larvae of some decapod species which are highly dependent on estuarine conditions were found only in the land-ward moving lower water strata while larvae of species not restricted to estuaries were found throughout the water column (Sandifer, 1975). The behaviors by which larvae actively participate in their transport are just now being described. Larvae could respond to numerous environmental cues to regulate their swim- ming activity. Thorson (1964) concluded that light is the main cue to swimming behavior and that changing responses to light can explain the observed ontogenetic differences in vertical distribution. The directional responses of crab larvae depend on light intensity, light adaptation, temperature, salinity and polarization. All crab 145 146 BULLETIN OF MARINE SCIENCE, VOL. 39, NO.2, 1986 larvae are sensitive to green light which maximally penetrates coastal waters (Forward and Cronin, 1978), while crabs from high intertidal habitats have high sensitivity to ultraviolet light (Forward and Cronin, 1979). Phyllosoma larvae of the lobster, Panu/irus longipes, exhibit additional maxima at 470 nm and 615 nm, probably because they are also adapted to oceanic waters with maximum transparency at 400-500 nm (Ritz, 1972). In general, all crab zoeal stages are photopositive (Forward and Costlow, 1974; Sulkin, 1975), while megalopas may be either photopositive (Sulkin, 1975; Bigford, 1979) or indifferent (Forward and Costlow, 1974). The larvae of the crab, Rhithropanopeus harrisii, exhibit complex vertical migration at four zoeal stages so that they would remain in the strata of least net flow thereby reducing their longitudinal transport (Cronin, 1982). Evi- dence for an endogenous rhythm in these behaviors also exists (Cronin and For- ward, 1979). It is not clear whether negative phototaxis or increased sinking rate is the mechanism by which larvae ready to settle seek the sea floor. For example, cyprids, but not nauplii, of barnacles cease swimming in light (Crisp and Ritz, 1973). Photonegative behavior may be most important at the very moment of settlement because it brings the larvae into cryptic and shaded habitats which could provide protection from silt, predation and other sources of mortality (Buss, 1979). Gravity is the most constant physical factor to which larvae can respond. Cen- trifugation experiments which exclude the effects of buoyancy, dissolved gases and pressure demonstrate direct, active orientation to gravity in larvae of the bryozoan Bugula stolonifera, while those of B. neritina possess some other means of maintaining a vertical orientation (Pires and Woollacott, 1983). Larvae less than 1 mm in length, a size at which viscous forces are more important than inertial ones, generally swim by means of cilia alone while larger larvae, at a size where inertial forces gain importance, can efficiently use muscular activity (Vogel, 1981). The relationship between viscosity and inertia (the Reyn- olds number) incorporates terms for the length oflarvae and the mean swimming velocity. At the low Reynolds numbers larvae experience, larval length not shape is the important morphological parameter. Thus the diverse morphologies of small invertebrate larvae do not improve swimming efficiency per se but rather have developed to place the cilia at efficient positions for swimming and feeding (Strath- mann, 1974b; Emlet, 1983; Chia et al., 1984). MacBride (1914) states that the larval phase of development represents a former condition of the adult in keeping with Haeckel's biogenetic law. Citing larval transparency, small size and specialized swimming structures, Garstang (1922) countered MacBride's ideas and stated that larvae were specially adapted for a planktonic existence. This last view has evolved into the thesis that larval forms are maintained under selection for dispersal. Strathmann (197 4a) pointed out that the spread of sibling larvae could confer short-term advantages to individuals by permitting the larvae to sample a number of independently varying habitats. These ideas were further pursued in a series of models which investigated the effects of scale of dispersal (Palmer and Strathmann, 1981). They showed that at larger scales an asymptote of maximum relative advantage is reached and furthermore, that the asymptote is higher and more slowly approached as increasing environ- mental variance is factored in. More recently Strathmann (1980) has expressed the opinion that no evidence exists to either support or refute the short-term advantage hypothesis. The larval planktonic period can be divided into two parts: a precompetent period before the larva is capable of settling and a competent period during which it is capable of settling if the appropriate environmental cues are encountered. A CAMERON: INTRODUCTION TO INVERTEBRATE WORKSHOP 147 mathematical model using eddy diffusion for larval dispersal predicts that the length of the precompetent and competent larval periods will covary and that the competent period will be equal to or greater than the precompetent period (Jackson and Strathmann, 1981). The scant data on these periods agree with the predictions. One optimization model for the duration of the competent period concerns avail- ability of favorable substrata and the balance of risks of settling at a suboptimal spot or returning to the plankton to search further (Doyle, 1975). Using examples from nudibranch molluscs, Todd and Doyle (1981) propose a settlement-timing hypothesis which states that planktotrophic larval strategies alone are of sufficient length to bridge the period between optimum spawning times and optimum set- tlement times. In subsequent discussions of this idea Hadfield and Switzer-Dunlap (1984) offer the alternate hypothesis that the broad reproductive season and large juvenile size are an adaptation to a variable food supply at settlement. In a theoretical treatment, Istock (1967) makes a case for viewing the larval phase as selected to exploit an alternate food source away from competition with adults. These alternative views propose a variety of selective forces other than dispersal which could account for the maintenance of a larval stage. The difficulty of tracking larval transport renders most conclusions about dis- persal debatable. Until the fate of discrete populations of larvae with known pedigrees can be described in time and space, the investigator is left with only partially reliable estimates on which to base predictions about population dy- namics and life history parameters. Settlement and Recruitment Many, if not all, benthic marine invertebrates show both temporal and spatial variation in recruitment. The study of the environmental factors that are the proximate causes for the observed variation has been a perennial activity of marine biologists (Thorson, 1950; Frank, 1975). The reasons for these investigations include practical
Details
-
File Typepdf
-
Upload Time-
-
Content LanguagesEnglish
-
Upload UserAnonymous/Not logged-in
-
File Pages17 Page
-
File Size-