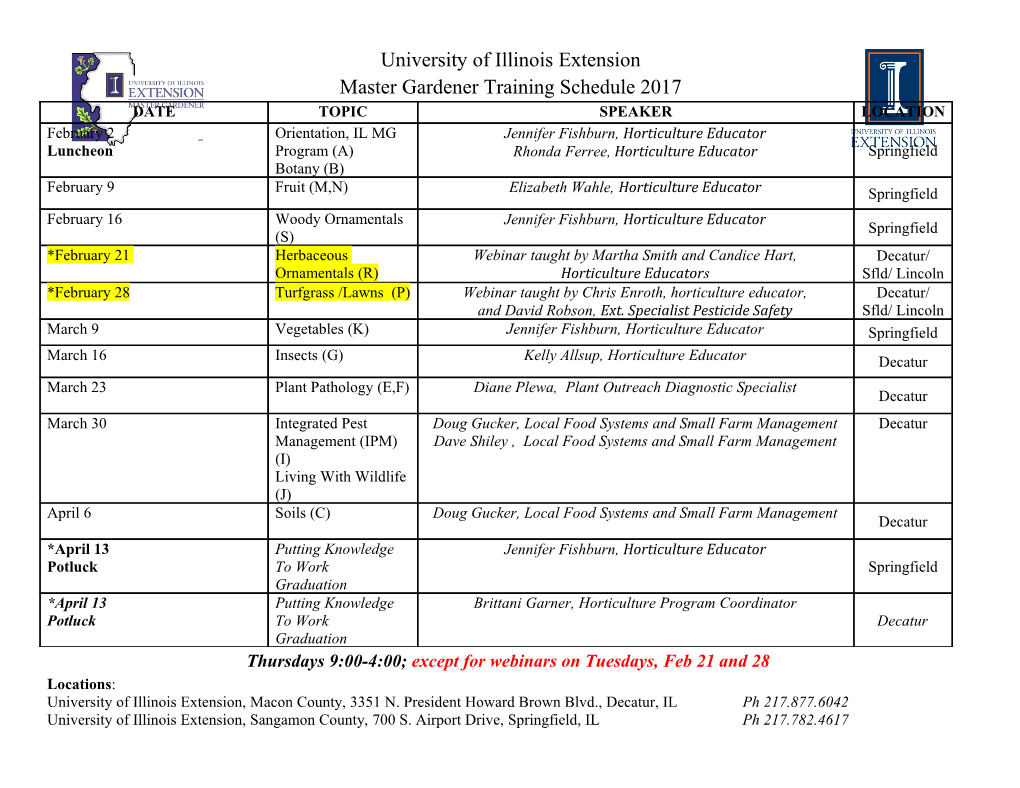
Oikos 119: 1862–1869, 2010 doi: 10.1111/j.1600-0706.2010.18782.x © 2010 Th e Authors. Oikos © 2010 Nordic Society Oikos Subject Editor: Jonathan W. Moore. Accepted 30 August 2010 A framework for understanding physical ecosystem engineering by organisms Clive G. Jones , Jorge L. Guti é rrez , James E. Byers , Jeffrey A. Crooks, John G. Lambrinos and Theresa S. Talley C. G. Jones ([email protected]), Cary Inst. of Ecosystem Studies, PO Box AB, Millbrook, NY 12545, USA; and Direction Scientifi que, AgroParisTech, 19 avenue du Maine, FR – 75732 Paris, France. – J. L. Guti é rrez, Grupo de Investigaci ó n y Educaci ó n en Temas Ambientales (GrIETA), Espa ñ a 3364, Mar del Plata (7600), Argentina; and Facultad de Ciencias Exactas y Naturales, Depto de Biolog í a, Univ. Nacional de Mar del Plata, Funes 3250, 2 ° Piso, Mar del Plata (7600), Argentina. – J. E. Byers, Odum School of Ecology, Univ. of Georgia, 140 E. Green St., Athens, GA 30602, USA. – J. A. Crooks, Tijuana River National Estuarine Research Reserve, 301 Caspian Way, Imperial Beach, CA 91932, USA. – J. G. Lambrinos, Dept of Horticulture, Oregon State Univ., 4017 Agriculture and Life Sciences Building, Corvallis, OR 97331-73044, USA. – T. S. Talley, Integrative Oceanography Division, Scripps Inst. of Oceanography, Univ. of California, La Jolla, CA 92093, USA . While well-recognized as an important kind of ecological interaction, physical ecosystem engineering by organisms is diverse with varied consequences, presenting challenges for developing and using general understanding. Th ere is also still some uncertainty as to what it is, and some skepticism that the diversity of engineering and its eff ects is amenable to conceptual integration and general understanding. What then, are the key cause/eff ect relationships and what underlies them? Here we develop, enrich and extend our extant understanding of physical ecosystem engineering into an integrated framework that exposes the essential cause/eff ect relationships, their underpinnings, and the interconnections that need to be understood to explain or predict engineering eff ects. Th e framework has four cause/eff ect relationships linking four components: 1. An engineer causes structural change; 2. Structural change causes abiotic change; 3. Structural and abiotic change cause biotic change; 4. Structural, abiotic and biotic change can feedback to the engineer. Th e fi rst two relationships describe an ecosys- tem engineering process and abiotic dynamics, while the second two describe biotic consequence for other species and the engineer. Th e four relationships can be parameterized and linked using time-indexed equations that describe engineered system dynamics. After describing the relationships we discuss the utility of the framework; how it might be enriched; and briefl y how it can be used to identify intersections of ecosystem engineering with fi elds outside ecology. Ecosystem engineering by organisms (Jones et al. 1994, (Jones et al. 1994, Berkenbusch and Rowden 2003, Wright 1997a) is now well-recognized as an important general kind and Jones 2006). Our objectives are to amplify and clarify of ecological interaction of basic and applied relevance (Crooks what physical engineering is, and show that the diversity of 2002, Rosemond and Anderson 2003, Boogert et al. 2006, cause and eff ects is amenable to general understanding. We Byers et al. 2006, Wright and Jones 2006, Cuddington et al. thereby hope to facilitate theory development and model- 2007). Yet ecosystem engineering is diverse with varied conse- ing, hypothesis formulation, comparison and generalization, quences, presenting challenges for developing general under- empirical study and methodological development in this standing from case studies that can then be applied to other rapidly growing fi eld of research. We also hope that by for- studies. Th ere is also still some uncertainty as to what enginee- mally exposing the relationships and their underpinnings, the ring is and is not; some skepticism that the diversity of engi- framework will help other fi elds such as geomorphology, envi- neering and its eff ects is amenable to conceptual integration ronmental engineering, and evolutionary biology, identify their and general understanding; and continued debate over the points of intersections with ecosystem engineering. value of the mechanistic approach that underlies the con- cept (Reichman and Seabloom 2002a, 2002b, Wilby 2002, Wright and Jones 2006, Cuddington 2007, Wilson 2007, Framework: engineering cause and Jones and Guti é rrez 2007). effect relationships Here we develop, enrich and extend our extant understand- ing of physical ecosystem engineering into an integrated frame- Four general cause and eff ect relationships link four compo- work that exposes the essential cause/eff ect relationships, their nents (Fig. 1): 1. An engineer causes structural change; 2. Struc- underpinnings, and the interconnections that need to be tural change causes abiotic change; 3. Structural and abiotic understood to explain or predict engineering eff ects, and change cause biotic change; 4. Structural, abiotic, and biotic that can help make sense of, and generalize from, case studies change can feedback to the engineer. Th e fi rst two relationships 1862 Table 1. Parameters and time (t) -indexed equations (magnitudes, rates, frequencies) for engineered system dynamics. As illustrated through- out the text, the general notations make no assumptions about the shapes of relationships; include the potential for fi rst or higher order interaction terms; allow one or more independent variables to have zero values if not relevant (e.g. B ϭ 0 and S ϭ 0 in Eq. 7 when there is only an abiotic feedback to engineer density); allow for substituation across equations (e.g. P and ρ for K, when an engineer generates kinetic energy); and can be specifi ed for particular study systems (e.g. inclusion of parameters describing the shape of the relationship, system-specifi c covariates and additional variables). S: Structural state (physical properties) F: Structure formation by engineer (construction and maintenance) D: Structural decay P: Per capita engineering activity ρ : Engineer density R: Intrinsic durability of structural materials Figure 1. Physical ecosystem engineering by organisms. Th e cause/ I: Intensity of structurally destructive forces A: Abiotic state (relevant abiotic variables) eff ect relationships representing an engineered system. Th e solid K: Kinetic energy (other than K generated by P, ρ ) arrow for autogenic engineering represents the physical manifesta- M: Abiotic materials (fl uids, solids) tion of organismal structure inserted into the abiotic milieu. Th e striped B: Biotic state as biotic response variables of interest arrow for allogenic engineering represents the action of the engineer Structural change on other living or non-living structure. ϭ ϩ St ϩ 1 St Ft – Dt … … … … … … … … … … … … Eq. 1 Where: describe an ecosystem engineering process and abiotic dynam- ϭ ρ Ft f (Pt ’ t ) … … … … ... … … … ...… … … … … … Eq. 2 ics, while the second two describe biotic consequence for other ϭ Dt f (Rt’ It ) … … … … … ... … … ...… … … … … … Eq. 3 ϭ species and the engineer (Jones and Guti é rrez 2007). All four S0 Baseline unmodifi ed structural state relationships may need to be interconnected to understand Abiotic change ϭ engineered system dynamics. Most of the sections below con- At ϩ 1 f (At ’ St ’ Kt ’ Mt ) … … … … ...… … … … … ... Eq. 4 ϭ tain an overview followed by more detailed explanations. A0 Baseline unmodifi ed abiotic state Biotic change ϭ Bt ϩ 1 f (Bt ’ St ’ At ) . … … … … … … … .… … … … … Eq. 5 Engineer causes structural change ϭ B0 Baseline unmodifi ed biotic state An engineer species autogenically and/or allogenically (Jones Engineering feedbacks P ϩ ϭ f (P S A B ) … … … … ... … …… … … … .. Eq. 6 et al. 1994) causes structure formation in the abiotic envi- t 1 t’ t’ t’ t ρ ϩ ϭ f ( ρ S A B ) … … … … … … … … … … … .. Eq. 7 ronment, creating a new structural state relative to a baseline, t 1 t’ t’ t’ t unmodifi ed state. Th e degree of structure formation via con- struction and maintenance is a function of per capita engineer- Many sessile organisms are obvious autogenic engineers (e.g. ing activity (hereafter activity) and engineer density (Jones trees). However, engineers can be mobile and autogenic (e.g. et al. 1994; Table 1, Eq. 2). For example, coral reefs grow; a shells of living crabs create epibiont habitat), sessile and allo- pair of beaver builds a dam. Unless maintained, engineered genic (e.g. plant root growth creates soil macropores), and structures, like all physical structures, undergo structural decay simultan eously auto- and allogenic (e.g. many plants, micro- toward a baseline structural state. Th eir persistence in the bial soil surface crusts). absence of maintenance is a function of the intrinsic durabil- Assigning an organism to one, the other, or both categories ity of the structural materials and the intensity of structurally is less important than what structural origins can reveal about destructive forces (Jones et al. 1997a; Table 1, Eq.3). Engineer constraints on construction and maintenance and the onset death (for autogenic) and structure abandonment (for allo- of structural legacies. Autogenically engineered structures will genic) therefore leave decaying structural legacies (Hastings form if the organism can grow in that environment. Con- et al. 2007). Structural change over time is thus the sum of struction and maintenance are
Details
-
File Typepdf
-
Upload Time-
-
Content LanguagesEnglish
-
Upload UserAnonymous/Not logged-in
-
File Pages8 Page
-
File Size-