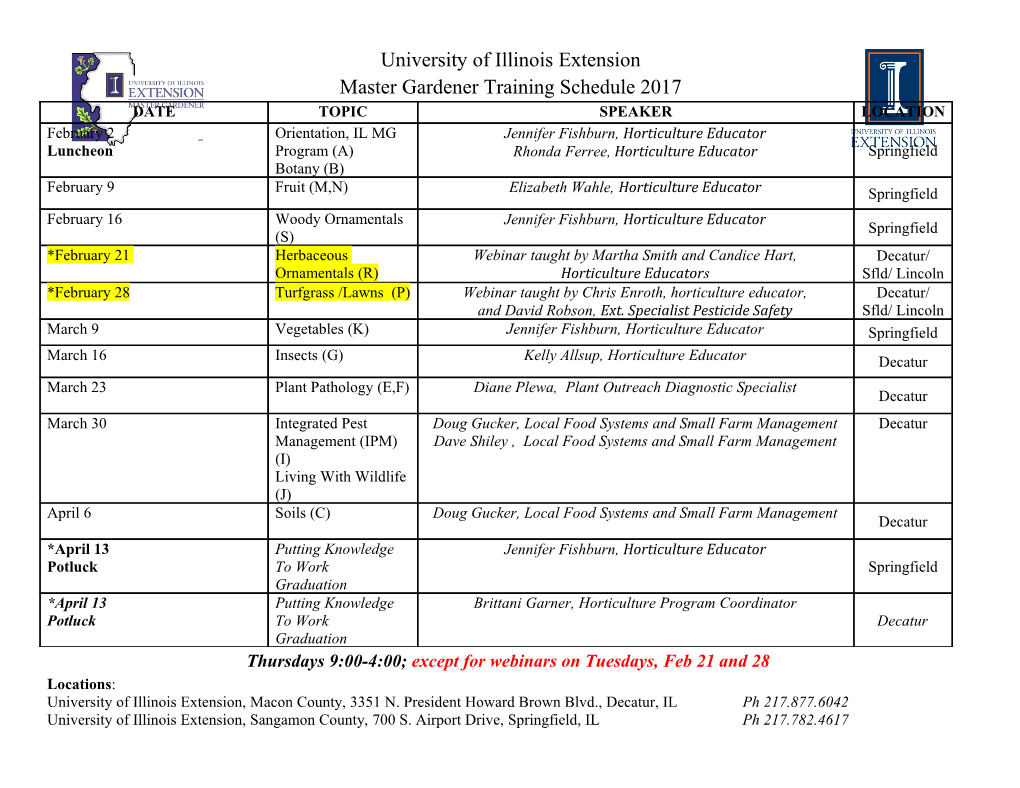
Chem 481 Lecture Material 3/13/09 Radiation Detection and Measurement When radiation interacts with matter electronic excitation and ionization occur. When de-excitation results in the emission of photons these can be detected by suitable light- sensitive devices. When charge carriers (ion pairs, electron/hole pairs) are produced these can be collected to generate an electrical signal. The dead time of a detector is the length of time between the formation of an output signal (pulse) and when the detector conditions are able to produce another output signal (see figure below). Initially this second output is insufficient in size to be recognized by the counting electronics (less than the discriminator level). The resolving time is the interval between the initial pulse and the formation of a detectable pulse (see figure below). The recovery time is the interval between two full-size pulses. Events that occur during the resolving time will not be recorded and are known as coincidence losses. Gas Ionization Detectors Imagine a gas-filled container with 2 electrodes of opposite charge (see figure below). As radiation enters the gas filling and ionizes the gas (creates primary cation-electron pairs), the cations will drift toward the negatively-charged electrode and the electrons Radiation Detection and Measurement 3/13/09 page 2 will be collected at the positive anode resulting in a current that can be measured. Some cations and electrons may recombine. As the electric potential across the electrodes is increased a voltage is reached where the electrode attraction is greater than the attraction between cations and electrons and all primary ion pairs are collected. Small increases in voltage beyond this continue to ensure collection of all primary ion pairs. The collected current depends on the number of primary ion pairs which depends on the specific ionization of the radiation. Thus, the output signal will be different for alpha, beta and gamma radiation. A gas-filled detector that operates at a voltage where all primary ion pairs are collected (saturation region) is called an ionization chamber (see figure below). Because there is a wide range of voltage associated with the saturation region, a well- regulated voltage supply is not needed. Also, the low voltage requirements allow for battery operation and portability. Air is often used for the gas filling. The small current or voltage that is produced often requires amplification using an electrometer. The quartz fiber dosimeter is an electroscope that operates on the same principles as an ionization chamber. As the potential is further increased between the electrodes the current collected increases because electrons produced in the primary ionization become energetic enough as they are drawn toward the anode to cause secondary ionization. This Radiation Detection and Measurement 3/13/09 page 3 increase in the number of electrons beyond that produced in primary ionization events is call gas amplification. In this voltage region the amount of gas amplification is constant for a given voltage. Thus, the collected current is proportional to the number of primary ionization events and the output signal will vary depending upon the type of radiation (α > β > γ - see figure above) and energy of the radiation. A gas-filled detector that operates at a voltage where there the gas amplification maintains a linear proportionality with the primary ionization is called a proportional counter. The proportionality of pulse height and primary ionization is maintained because gas amplification (Townsend avalanche) is confined to a very small distance (0.01 mm) from a fine wire anode (see figure below). Since only the electrons are collected for the output signal, the output pulse has a fast rise time and the detector has a short dead time (and short resolving time). This means that proportional counters can handle higher counting rates before coincidence losses become significant. A proportional counter also produces larger output signals that requires less amplification, but requires a well-regulated high voltage supply. Since the pulse height is proportional to the extent of primary ionization, alpha radiation will produce a pulse that can be passed by the discriminator at a lower gas amplification, and hence lower applied voltage, than will beta radiation. This means that for a source emitting both alpha and beta radiation, a plot of count rate versus applied voltage will exhibit 2 plateaus, first one produced by the alpha radiation and then a second one due to both the alpha and beta radiation (see figure below). Radiation Detection and Measurement 3/13/09 page 4 The operating characteristics of a proportional counter depend on its construction (size, shape, geometry of gas volume and electrodes) and on the type of gas filling. A common filling gas is a mix of 90% Ar and 10% CH4 (P-10 gas). The methane acts as quenching agent. It restricts the extent of the Townsend avalanche by removing energy from excited argon ions and then de-excites by dissociation instead of emitting photons that can trigger secondary avalanches. Air and water vapor should not be present because they attract electrons to form negative ions which will affect the output pulse size. A windowless counter for alpha and low-energy beta radiation positions the sample within the gas filling (see figure below). Nearly 2π counting geometry is achieved this way. As the applied voltage is increased further there is an increase in the gas amplification, but there is a limit based on the construction of the counter and gas filling. At some point the collected current is no longer proportional to the primary ionziation (limited proportional region). If the voltage is further increased eventually all radiation, regardless of the number of primary ionization events, produce the same size output pulse. This is known as the Geiger region and gas-filled detectors operating in this region are known as Geiger-Müller (G-M) counters (see Figure 8.2 on p.2). G-M counters operate where there is maximum gas amplification and the pulse amplitude is independent of the primary ionization. The amplification results from a Townsend avalanche that is propagated through the gas filling by UV photons emitted from excited atoms/ions (see figure below). These photons produce photoelectrons at other points in the gas filling until the build-up of a positive ion sheath reduces the electric field strength sufficiently to prevent further ionization. In order for the detector to again produce detectable output pulses, this extensive positive ion sheath must migrate to the cathode, a process that can take 100-300 microseconds. Thus, G-M counters have a much longer resolving time than proportional counters and coincidence Radiation Detection and Measurement 3/13/09 page 5 losses become significant at count rates below 10000 cpm. G-M cannot distinguish between different types of radiation, however, output pulse size is much larger so electronic amplification is usually unnecessary. As the positive ion sheath nears the cathode and cations become neutralized, the excited atoms can emit electrons or photons that can re-trigger the detector. This is prevented by adding a small amount of an organic compound or halogen to serve as a quenching agent. The noble gas ion transfers its charge to the molecule which gains an electron at the cathode. The energy acquired by neutralization is dissipated by dissociation rather than photon or electron emission. .
Details
-
File Typepdf
-
Upload Time-
-
Content LanguagesEnglish
-
Upload UserAnonymous/Not logged-in
-
File Pages5 Page
-
File Size-