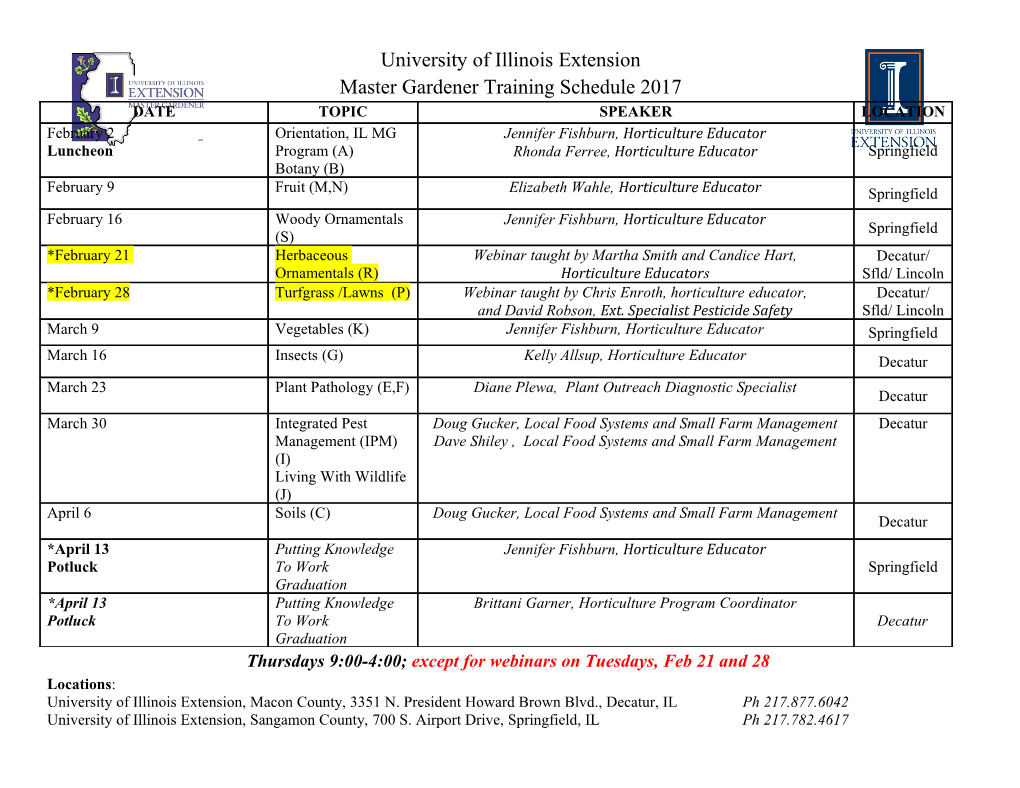
Draft version April 10, 2018 A Preprint typeset using LTEX style emulateapj v. 01/23/15 DIRECT MEASURE OF RADIATIVE AND DYNAMICAL PROPERTIES OF AN EXOPLANET ATMOSPHERE Julien de Wit1, Nikole K. Lewis2, Jonathan Langton3, Gregory Laughlin4, Drake Deming5, Konstantin Batygin6, Jonathan J. Fortney4 Draft version April 10, 2018 ABSTRACT Two decades after the discovery of 51 Peg b, the formation processes and atmospheres of short-period gas giants remain poorly understood. Observations of eccentric systems provide key insights on those topics as they can illuminate how a planet’s atmosphere responds to changes in incident flux. We report here the analysis of multi-day multi-channel photometry of the eccentric (e ∼ 0.93) hot Jupiter HD 80606 b obtained with the Spitzer Space Telescope. The planet’s extreme eccentricity combined with the long coverage and exquisite precision of new periastron-passage observations allow us to break the degeneracy between the radiative and dynamical timescales of HD 80606 b’s atmosphere and constrain its global thermal response. Our analysis reveals that the atmospheric layers probed heat rapidly (∼ 4-hr radiative timescale) from < 500K to 1400K as they absorb ∼ 20% of the incoming +85 stellar flux during the periastron passage, while the planet’s rotation period is 93−35 hours, which exceeds the predicted pseudo-synchronous period (40 hours). Keywords: planet-star interactions, planets and satellites: atmospheres, planets and satellites: dynam- ical evolution and stability, planets and satellites: individual: HD 80606 b, techniques: photometric, methods: numerical 1. INTRODUCTION As the planet’s orbital period decreases, the Kozai os- The discovery of 51 Peg b (Mayor & Queloz 1995), the cillations are damped by general relativistic precession first extrasolar planet orbiting a sun-like star, was an and other effects of comparable magnitude, marooning epoch-making event. The now-famous 51 Peg b, which the planet on an inclined, gradually circularizing orbit. When orbital circularization is complete, the end prod- has P = 4.23 d and M sin(i)=0.47MJup, rapidly be- came the prototype hot Jupiter—gas giants on short- uct is a hot Jupiter in an orbit that is misaligned with period orbits. In the past two decades, a substantial the equator of the parent star. number of such planets have been observed and stud- The HD 80606 system (Naefetal. 2001; ied along their orbits (Charbonneau et al. 2002, 2005; Laughlin et al. 2009; Moutou et al. 2009), with its Deming et al. 2005; Knutson et al. 2007). The existence V=8.93 G5V primary and M=3.94 MJup gas giant of hot Jupiters challenged standard planetary system for- planet, appears to be an excellent example of a planet mation and evolution theories developed in the context embarking on the final circularizing phase of the KCTF of our own solar system. process. The external solar-type binary perturbing star, HD 80607, is plainly visible at a projected sep- The mechanism of Kozai cycles with tidal fric- ∼ tion, or KCTF (Eggleton & Kiseleva-Eggleton 2001; aration of 1000 AU. The planet HD 80606 b, with a P =111.43637d orbit, has an extremely high orbital Wu & Murray 2003; Fabrycky & Tremaine 2007), is an ◦ ± ◦ attractive process to explain the current orbits of many eccentricity (e = 0.93366) and a λ = 42 8 sky- of the known hot Jupiters. KCTF occur when a dis- projected misalignment between the orbital and stellar tant, sufficiently inclined stellar binary or planetary spin angular momentum vectors (Moutou et al. 2009; companion forces a planet on an initially long-period Winn et al. 2009), and experiences strong, episodic radiative and tidal forcing as a result of periastron arXiv:1606.01105v1 [astro-ph.EP] 3 Jun 2016 low-eccentricity (e < 0.1) orbit to experience periodic episodes of very high eccentricity (e > 0.5), a process encounters that sweep the planet a mere 6R⊙ from the known as Kozai cycling (Kozai 1962; Lidov 1962). Dur- stellar surface (the geometry of the system is indicated ing a planet’s closest approach to its host star, periastron in Figure 1). passage, tidal dissipation converts orbital energy into Weather variations in the atmosphere of HD 80606 b heat, thereby decreasing the planet’s semi-major axis. are naturally expected to be extreme. The flux received by the planet intensifies by a factor f = ((1 + e)/(1 − e))2 = 850 between apoastron and periastron, with the 1 Department of Earth, Atmospheric and Planetary Sciences, MIT, 77 Massachusetts Avenue, Cambridge, MA 02139, USA major fraction of this increase coming during the 24-hour 2 Space Telescope Science Institute, 3700 San Martin Drive, period prior to the periastron passage. The large, impul- Baltimore, MD 21218, USA 3 sive deposition of energy into the planetary atmosphere Department of Physics, Principia College, Elsah, IL 62028, (equivalent to ∼1000x the energy delivered to Jupiter by USA 4 Department of Astronomy and Astrophysics, University of the Shoemaker-Levy impacts) drives a global tempera- California, Santa Cruz, CA 95064, USA ture increase that we have observed with Spitzer Space 5 Department of Astronomy, University of Maryland at Col- Telescope’s IRAC detector (Werner et al. 2004). lege Park, College Park, MD 20742, USA 6 Division of Geological and Planetary Sciences, California In- stitute of Technology, Pasadena, CA 91125, USA 2. OBSERVATIONS AND DATA REDUCTION 2 de Wit et al. Using the 4.5-µm channel of the IRAC detector in sub- for these instrumental systematics using standard tech- array (32×32 pixel) mode, we obtained a series of 676,000 niques described below allowing us to reach photometric photometric measurements of HD 80606 in two successive precisions of 55 and 100 ppm per 1-hr bin at 4.5 and blocks of 50 and 30 hrs starting on UT Jan 06 2010 and 8 µm, respectively. The resulting 0.1% flux variation of ending on Jan 09 2010, with a 0.4 sec exposure time. the system during the course of the observations is shown The first block started 34 hours before the periastron in Figure 2. passage at HJD 2455204.91. The second block started We analyze HD 80606’s photometry using a method after a three-hour interruption for data transfer follow- previously introduced in the literature (de Wit et al. ing the acquisition of the first block. In addition, we have 2012; de Wit 2015). The method is implemented as an re-analyzed a separate (shorter) set of photometric mea- adaptive Markov Chain Monte Carlo (MCMC) (Gregory surements obtained in 2007 using Spitzer’s 8-µm channel 2005; Ford 2006) algorithm, allowing us to stochastically (Laughlin et al. 2009). sample the posterior probability distribution for our cho- sen model, providing an estimate of the best-fit model 2.1. Spitzer 4.5-µm Photometry parameters, their uncertainties, and any covariances be- We calculate the time at mid-exposure for each image tween parameters. We update the method to include the from time stamps stored in the FITS header. For each transient heating of the planet’s atmosphere due to its FITS file, we determine the background level of each of eccentric orbit. the 64 subarray images from the pixels located outside a 3.1. Nominal Model 10 pixel radius, excluding any flagged hot,bright or pe- ripheral pixels. Using a histogram of the measured back- We use a simple energy-conserving model of the ground pixel values we discard the 3σ outliers from the planet’s global atmospheric response to estimate the at- background pixels. We estimate the background value mospheric properties of HD 80606 b by fitting the time based on the median of the undiscarded background pix- series data. The model assumes that the photometry els. We then subtract this background value from each is unaffected by any advective hydrodynamical response, image. From the background subtracted image, we de- and that the planet has a uniform global temperature, T0, termine the stellar centroid based on a flux-weighted cen- 50 hrs prior to its periastron passage, which is consistent troid method using a circular aperture with a radius of with predictions from global circulation models for exo- 4 pixels (Knutson et al. 2008; Lewis et al. 2013). We es- planets on high eccentricity orbits (Langton & Laughlin timate the stellar flux from each background subtracted 2008; Kataria et al. 2013; Lewis et al. 2014). For both image using a circular aperture centered on the estimated the 4.5-µm and the 8-µm channels, at each point on a position of the star. We optimize our choice of aperture planetary latitude (θ) longitude (φ) grid, and at each individually for each AOR looking both at the white and time stamp, we compute the rate of temperature change red noise contributions for time-varying and fixed aper- T˙ using tures (Knutson et al. 2012; Lewis et al. 2013). We find ˙ 4 − 4 T = γT (Teq T ), (1) that for all the AORs time-varying apertures equal to the square root of the noise pixel parameter β˜ with a con- where γT is a parameter related to the radiative response stant offset minimize the scatter and the relative amount rate and Teq(t,φ,θ) is the temporally- and spatially- of red noise in the final time series and have an average varying equilibrium temperature of the atmosphere. aperture of 1.8 pixels. Specifically, we take Finally, we remove outliers from our final time series 4 L∗ 4 sets using 4 σ moving filters with widths of 64, 1000, Teq = f 2 cos α + T0 . (2) 2000, and 4000 on the photometry and the centroid po- 4πσr sition. The use of multiple large filters allow us to re- In the above equation, the instantaneous star-planet move pointing excursions with longer (∼ 1 minute) du- distance, r, is r = a(1 − e cos E), where a is the semi- rations, such as those due to micrometeorite impacts on major axis and E is the eccentric anomaly, which is re- the spacecraft.
Details
-
File Typepdf
-
Upload Time-
-
Content LanguagesEnglish
-
Upload UserAnonymous/Not logged-in
-
File Pages8 Page
-
File Size-