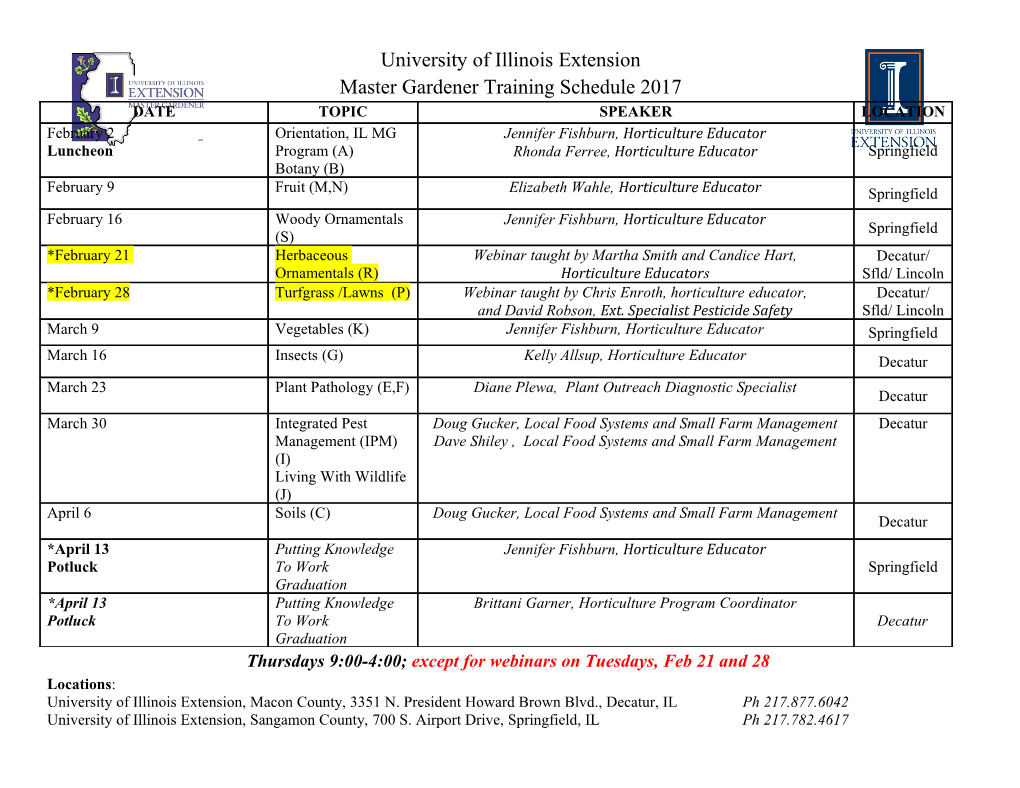
crystals Review Can Microbially Induced Calcite Precipitation (MICP) through a Ureolytic Pathway Be Successfully Applied for Removing Heavy Metals from Wastewaters? Álvaro Esteban Torres-Aravena 1,2,* , Carla Duarte-Nass 2, Laura Azócar 3, Rodrigo Mella-Herrera 2, Mariella Rivas 4,5 and David Jeison 6 1 Departamento de Ingeniería Química, Universidad de La Frontera, Temuco 4780000, Chile 2 Núcleo Científico y Tecnológico de Biorecursos (BIOREN), Universidad de La Frontera, Temuco 4780000, Chile; [email protected] (C.D.-N.); [email protected] (R.M.-H.) 3 Departamento de Química Ambiental, Facultad de Ciencias, Universidad Católica de la Santísima Concepción, Concepción 4090541, Chile; [email protected] 4 Centro de Investigación Científico Tecnológico para la Minería CICITEM, Antofagasta 1240000, Chile; [email protected] 5 Laboratorio de Biotecnología Algal y Sustentabilidad, Facultad de Ciencias del Mar y recursos Biológicos, Universidad de Antofagasta, Antofagasta 1240000, Chile 6 Escuela de Ingeniería Bioquímica, Facultad de Ingeniería, Pontificia Universidad Católica de Valparaíso, Valparaíso 2362803, Chile; [email protected] * Correspondence: [email protected] Received: 11 October 2018; Accepted: 2 November 2018; Published: 21 November 2018 Abstract: Microbially induced calcite precipitation (MICP) through a ureolytic pathway is a process that promotes calcite precipitation as a result of the urease enzymatic activity of several microorganisms. It has been studied for different technological applications, such as soil bio-consolidation, bio-cementation, CO2 sequestration, among others. Recently, this process has been proposed as a possible process for removing heavy metals from contaminated soils. However, no research has been reported dealing with the MICP process for heavy metal removal from wastewater/waters. This (re)view proposes to consider to such possibility. The main characteristics of MICP are presented and discussed. The precipitation of heavy metals contained in wastewaters/waters via MICP is exanimated based on process characteristics. Moreover, challenges for its successful implementation are discussed, such as the heavy metal tolerance of inoculum, ammonium release as product of urea hydrolysis, and so on. A semi-continuous operation in two steps (cell growth and bio-precipitation) is proposed. Finally, the wastewater from some typical industries releasing heavy metals are examined, discussing the technical barriers and feasibility. Keywords: microbially induced calcite precipitation (MICP); heavy metals; wastewater treatment; bioprecipitation; calcium carbonate 1. Heavy Metals and Environmental Problems The contamination of watercourses by heavy metals is a serious environmental problem that has increased because of rapid industrial development. Indeed, several economic activities, such as metal plating, galvanization, the extraction and processing of minerals, tanning, battery production, paper manufacture, and pesticide synthesis, generate wastewaters that can contain pollutants [1]. Many of these metals are micronutrients, that is, they are essential for cell growth [2]. However, at high concentrations, they may turn toxic or carcinogenic, causing serious health problems. Moreover, when entering the food chain, these can accumulate in the human body [3]. In this sense, special attention has Crystals 2018, 8, 438; doi:10.3390/cryst8110438 www.mdpi.com/journal/crystals Crystals 2018, 8, 438 2 of 13 been paid to the most hazardous pollutants, such as zinc, copper, nickel, mercury, cadmium, lead, and chromium [4]. Their adverse effect on natural and human environments demands the development of efficient and cost-effective technologies in order to ensure the removal of these heavy metals from the environment. 2. Conventional Treatment of Wastewaters Containing Heavy Metals Nowadays, several processes such as flotation, chemical precipitation, adsorption, ion exchange, membrane filtration, coagulation, and electrochemical deposition are available to treat metal-containing water [1,3]. Although these processes can remove metals efficiently (metal removal exceeding 90%) [1], their main constraints are associated with high energy requirements, the use of chemicals, and the production of toxic metal sludge. All of these characteristics contribute to increase overall costs [5,6]. In recent years, biological processes have been proposed and developed for metal removal from waters and wastewaters, for example bio-sorption, bio-accumulation, phytoremediation, bio-coagulation, bio-leaching, and application of sulfate-reducing bacteria (SRB) [6,7]. These biological processes have relevant advantages when compared with their conventional counterparts, such as reduced energy and material consumption, possibilities for metal recycling or recovery, and lower sludge production [8]. These characteristics transform them into potentially more eco-friendly alternatives. However, there are disadvantages mainly related to the final waste disposal, which is often a metal-containing biomass [9]. Moreover, the bacterial immobilization of metals may not constitute a long-term solution, when it is the result of changes in the redox state, as conditions in the environment may re-mobilize them [10]. 3. Microbially Induced Calcite Precipitation (MICP) Process 3.1. Precipitation by Ureolytic MICP Process MICP is a biological process in which calcite (CaCO3) formation is achieved as a result of the active metabolism of bacteria, which generates a favorable micro-environment for precipitation [11,12]. In calcite formation in the MICP through ureolytic pathway, bacteria catalyze urea hydrolysis into carbonate and ammonium. The latter produces an alkalization of the micro-environment, favoring the binding of calcium and carbonate, and furthermore, calcite precipitation [13,14]. Moreover, bacteria also provide nucleation sites in which the calcite precipitation takes place. Four steps can be identified for biological calcite precipitation, as illustrated in Figure1. (a) Urea hydrolysis: The urease enzyme hydrolyzes urea into carbamic acid and ammonia (Equation (1)). Furthermore, a spontaneous chemical equilibrium takes place and carbamic acid is converted into carbonic acid and ammonia (Equation (2)) [10,15]. Urease CO(NH2)2 + H2O ! NH2COOH + NH3 (1) Spontaneous NH2COOH + H2O ! H2CO3 + NH3 (2) (b) Chemical equilibrium: Ammonia from the urea hydrolysis turns into ammonium, releasing hydroxide ions and increasing the micro-environmental pH, which generates favorable conditions for further precipitation [11] (Equation (3)). Hydroxide ions induce carbonate formation from carbonic acid (Equations (4) and (5)). + − 2NH3 + 2H2O $ 2NH4 + 2OH (3) − + H2CO3 $ HCO3 + H (4) − + − 2− HCO3 + H + 2OH $ CO3 + 2H2O (5) Crystals 2018, 8, 438 3 of 13 (c) Heterogeneous nucleation: Calcium ions are bound to the external cell surface because of the negatively charged functional groups in the cell wall (Equation (6)). Then, calcite formation occurs in the cell surface, once the calcium ion activity is sufficient and the saturation conditions areCrystals favorable 2018, 8, x FOR for PEER CaCO REVIEW3 precipitation (Equation (7)) [16]. 3 of 13 occurs in the cell surface, once theCa calcium2+ + Cell ion activity! Ca 2is+ sufficient− Cell and the saturation conditions (6) are favorable for CaCO3 precipitation (Equation (7)) [16]. 2+ 2− Ca Ca− Cell+Cell+ CO 3Ca !CellCell − CaCO3 (6) (7) (d) Successive stratification: SuccessiveCa Cell+CO calcite layers Cell are CaCO developed on the external cell(7) surface (stratification)(d) Successive [stratification:17]. The nutrients Successive transfer calcite layers is limited, are devel andoped the on cells the get external embedded cell surface by calcite crystals,(stratification) provoking [17]. cellular The nutrients death. transfer is limited, and the cells get embedded by calcite crystals, provoking cellular death. FigureFigure 1. Schematic 1. Schematic illustration illustration ofof calcitecalcite productionproduction through through the the microbially microbially induced induced calcite calcite precipitationprecipitation (MICP) (MICP) process: process: 1—urea 1—urea hydrolysis;hydrolysis; 2—chemical 2—chemical equilibrium; equilibrium; 3—heterogeneous 3—heterogeneous nucleation;nucleation; 4—successive 4—successive stratification. stratification. Crystals 2018, 8, 438 4 of 13 Calcite precipitation through MICP has been regarded as a promising technology for different applications, such as for the improvement of mechanical properties in soils (bio-consolidation) [18,19], bio-cementation [14,20], crack repair in concrete structures [21], CO2 sequestration [22], bio-composites [23], hydraulic control [10], and so on. For a detailed review about the engineering applications of the MICP process, the authors recommend viewing the following literature [10,19]. 3.2. Factors Governing the Ureolytic MICP Process Several factors govern the ureolytic MICP process, such as the bacteria type, urea and Ca2+ concentrations, nucleation sites, pH, and temperature. Several microorganisms presenting high levels of urease activity have been identified, such as Sporosarcina pasteurii, Bacillus spCR2, Lysinibacillus sphaericus CH5, Bacillus pasteurii NCIM 2477, Kocuria flava CR1, Bacillus megaterium SS3, Bacillus thuringiensis, and Halomonas ssp. [24]. S. pasteurii is a non-pathogenic bacterium that is able to tolerate extreme conditions [24], and is probably the most common species used for testing and studying
Details
-
File Typepdf
-
Upload Time-
-
Content LanguagesEnglish
-
Upload UserAnonymous/Not logged-in
-
File Pages13 Page
-
File Size-