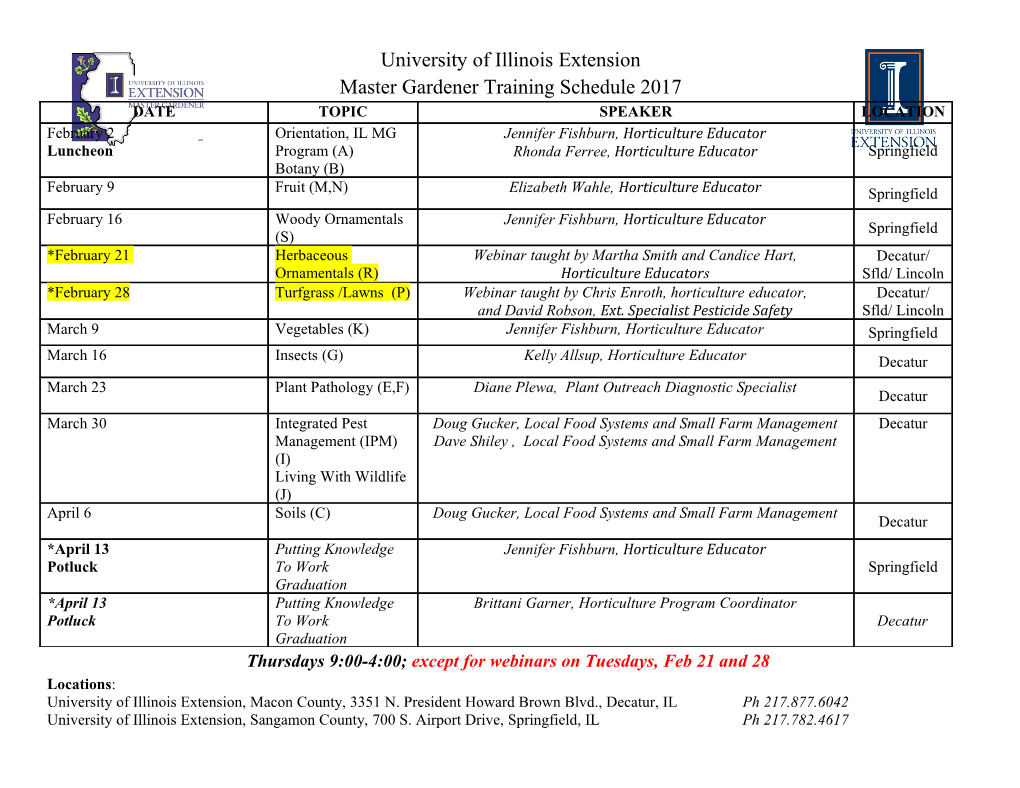
Robotic Developments for Extreme Environments – Deep Sea and Earth’s Moon B. Schäfer*, J. Albiez**, M. Hellerer*, M. Knapmeyer***, G. Meinecke****, O. Pfannkuche*****, L. Thomsen******, M. Wilde*******, T. Wimböck*, T. van Zoest******** *German Aerospace Center (DLR), Robotics and Mechatronics Center, Wessling, Germany e-mail: [email protected], [email protected], [email protected] **DFKI GmbH – Robotics Innovation Center (RIC), Bremen, Germany e-mail: [email protected] ***German Aerospace Center (DLR), Institute of Planetary Research, Berlin, Germany e-mail: [email protected] ****MARUM – Center for Marine Environmental Sciences, Bremen, Germany e-mail: [email protected] *****GEOMAR – Helmholtz Center for Ocean Research, Kiel, Germany e-mail: [email protected] ******Jacobs University Bremen (JUB), Bremen, Germany e-mail: [email protected] *******AWI – Alfred-Wegener-Institute, Polar and Marine Research, Bremerhaven, Germany e-mail: [email protected] ********German Aerospace Center (DLR), Institute of Space Systems, Bremen, Germany e-mail: [email protected] Abstract gies to improve the exploration of areas with extreme environmental conditions such as the deep sea, polar re- Robotic Exploration of Extreme Environments gions, and Earth's moon surface. This is a real unique (ROBEX) is a nationally funded Helmholtz alliance pro- endeavour to bring two different worlds together and to ject. It brings together space and deep-sea research insti- benefit from each other’s technological developments. tutions. The project partners are jointly developing tech- These technologies consider robotic developments and nologies for the exploration of highly inaccessible terrain, autonomous operations in deep underwater areas and on such as the deep sea and polar regions, as well as the Moon’s surface. The main idea behind is the develop- Moon and other planets. In order to increase the science ment of advanced exploration scenarios and finally its return from robotic systems used for exploration, more realization by demo-missions on Earth on relevant ana- sophisticated designs are needed which go beyond the logue sites. For that we wish to learn and benefit from present state-of-the-art. Advanced exploration scenarios the experience and expertise of each of the partners of are defined that finally will lead to demonstration mis- the two communities. This research alliance is config- sions on Earth analogue sites. Both communities expect ured to last for at least five years. In the meanwhile, we to highly benefit from each others developments, exper- have learned about the problems, further R&D desires tise and maturity of existing robotic systems, and those and existing solutions of different maturity levels in the being under development. two extreme areas. Autonomous operations, mobility and manipulabil- 1 Introduction ity are the three key issues to be covered. Long-range and long-time exploration of both extreme environments, Since the end of 2012, fifteen institutions distrib- in deep sea and on Earth’s Moon, require advanced mo- uted all over Germany are jointly developing technolo- bile systems in combination with skilled robotic arms that operate almost or fully autonomously [1]. In be established that gives rise to future increase of the ex- deep-sea applications several mobile systems, Autono- isting robotic capabilities. The common scenario will mous Underwater Vehicles (AUVs, Figure 1) and Re- foresee the exploration of larger areas of the seafloor and motely Operated Vehicles (ROVs, Figure 2), are already on the Moon surface, almost autonomously, by specific successfully in operation for many years, although most- scientific instruments that are transported by means of ly with very limited autonomous capabilities [2,3,4]. In vehicles and handled by robotic arms and versatile grip- space, the use of mobile systems is very rare, obviously pers. because of minor launch opportunities and high launch These two scenarios will be described in more de- and development costs. However, due to lack of direct tail hereafter. Both Moon and deep-sea scenarios will be and immediate in-situ involvement by humans, space studied and developed in two ways in parallel: one en- robotic systems benefit from their highly developed and visages an ideal scenario that aims to acquire and realize redundant components and increased autonomy in order the overall system more or less in a visionary manner, to operate without permanent human interaction [1,5]. but with regard to potential hardware and software con- straints. The second scenario then will be derived from the ideal one while slimming and reducing visionary ideas in order to achieve a realizable demo-mission here on Earth within the timeframe given for the ROBEX Al- liance project. Apart from the work on the specific sce- narios, further robotic study topics have been identified that will be investigated within certain design teams con- sisting of experts from all the topics. Those teams will study relevant robotic and mechatronic problem areas such as: tele-operated handling, camera guided intelli- gent grappler, docking interfaces, crawler (Figure 3) au- tonomy, object handling, underwater glider, legged crawler, and underwater navigation. Figure 1. AUV Abyss (GEOMAR) Figure 3. Deep-sea crawler in operation with data and power umbilical 2 Commonalities and Differences 2.1 General overview Exploration of deep sea and lunar environments re- quires un-manned mobile and manipulative systems that Figure 2. ROV PHOCA with 2 robotic arms are able to be tele-operated or even operated in semi- or Having all this in mind, the cooperation of the two fully autonomous modes. By nature, deep-sea applica- extreme exploration areas is expected to identify indi- tions are much more advanced compared to space appli- vidual and also common problem areas and to solve them cations. However, a limited number of such systems are by benefitting from each other. To start the cooperative already operating quite successfully on Mars. High mo- work, a common and typical exploration scenario has to bility, precise and skilled manipulability and autonomous operations of the robotic systems at very remote sites are required sub-components for the specific scenarios. For therefore important for successful exploration. In today´s robotics and mechatronics engineers in space applica- world robotic systems already explore the deep sea as tions, the distinction in the 3 major fields seems obvious: ROVs and AUVs, gliders and rovers/crawlers from ships, mobility, manipulation and autonomy. However, overlaps or even as components of a cabled observatory. are inherent and desired, e.g. in autonomous robotic arm To reach the goal of autonomous robotic exploration operations. In space, we mostly follow this (smart) dis- in both extreme environments while taking benefit from tinction. In deep sea, systems often are procured as a each other, we have to identify commonalities w.r.t. whole, whereas mobility, manipulation and some level of technological solutions, identify gaps in deep sea and autonomy is included in the delivery by the supplier. space technology, create operational (pilot) scenarios Moreover, the procured systems mostly allow some given the scientific needs, and finally define and develop flexibility by adding own solutions. Table 1. Common and different features in deep sea and space exploration missions Feature Deep Sea Earth’s Moon environment physi- high pressure, ~ 600 bar (6 km depth), high drag no pressure, no drag (vacuum) cal salty water no atmosphere almost no radiation solar wind / radiation (high energy radiation, particles, …) almost constant temperature around/above zero extreme temp. differences: -160 … +130 deg deg buoyancy vacuum visibility poor, only for short range visibility excellent, except shadowed areas and bright sunlight no static environment: moving particles, floating static environment: no dust, no storms, etc. objects, ocean currents terrain unknown, uneven on seafloor terrain unknown and uneven low gravity (1/6 g) slow Moon rotation (1/28 of Earth rotation) communication via cable cables not preferred acoustic waves (sonar) electromagnetic waves (RF) almost no time delay time delay power power cables solar power (solar panels) batteries secondary batteries (RTGs) autonomous opera- almost no time delay: tele-operation possible large time delay: tele-operation / direct control tions direct intervention possible (from ship) au- almost impossible tonomy less required no direct intervention autonomy required redundancy low, due to direct intervention for repair or change high, due to fail-safe demands and no direct intervention for change availability of oper- high (AUVs, ROVs, else), long tradition of usage extremely low, due to little launch opportuni- ational systems ties and high costs, applications are largely missing complexity of sys- medium to big very big tems mass no big issue light-weight structures etc. a must, due to launch impacts frame structure big issue, has to withstand high water pressure light-weight required, has to withstand launch loads system intelligence medium very high, due to autonomy and self-diagnosis demands 2.2 Common and different features reasons. The ROBEX project then will be finalized with the demonstration mission on Earth analogue sites, while Due to the very different environmental conditions, it putting several of these existing and modified hardware seems that differences are quite more prevailing than equipments
Details
-
File Typepdf
-
Upload Time-
-
Content LanguagesEnglish
-
Upload UserAnonymous/Not logged-in
-
File Pages10 Page
-
File Size-