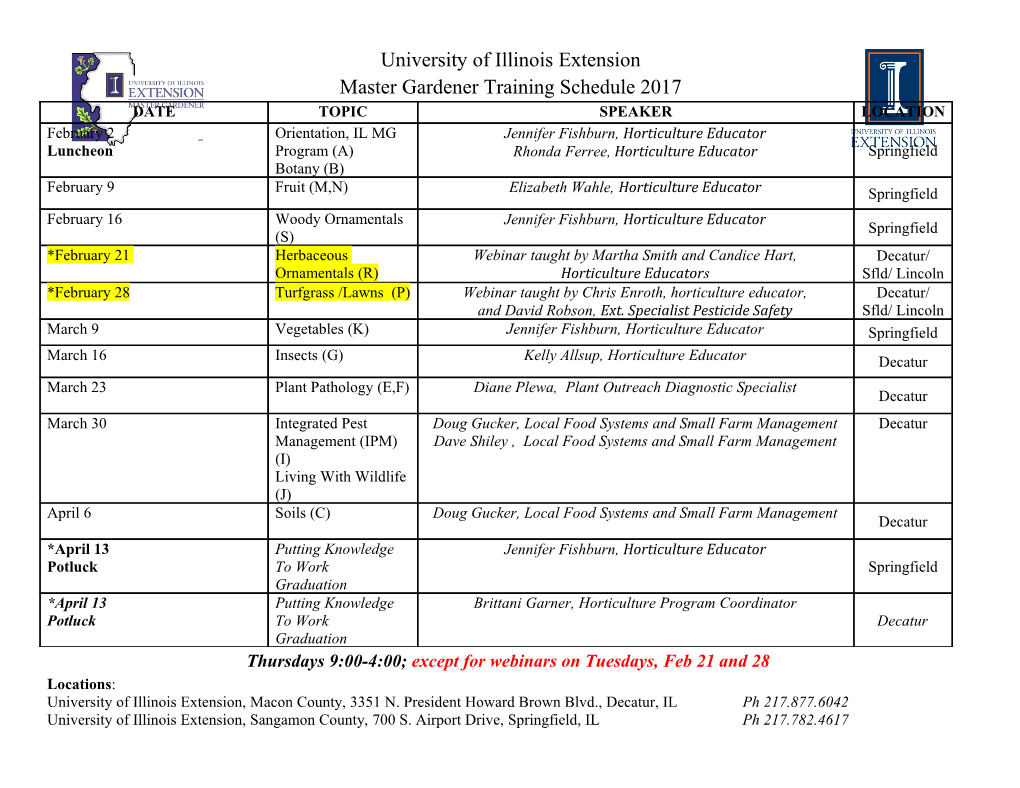
CPES ANNUAL REPORT 2020 103 Power Management Consortium (PMC) Nuggets 104 Low Loss Integrated Inductor and Transformer Structure and Application 114 Design Optimization of an Unregulated LLC Converter with Integrated in Regulated LLC Converter for 48 V Bus Converter Magnetics for a Two-Stage, 48 V VRM 105 Magnetic Integration of Matrix Transformer with a Highly Controllable 115 Wide-Voltage Range, High-Efficiency Sigma Converter 48 V VRM with Leakage Inductance Integrated Magnetics 106 Control Technique for CRM-Based, High-Frequency, 116 Modeling and Control for a 48 V/1 V Sigma Converter for Very Fast Soft-Switching Three-Phase Inverter Under Grid Fault Condition Transient Response 107 Critical Conduction Mode-Based, High-Frequency, Single-Phase 117 A Two-Stage Rail Grade DC-DC Converter Based on a GaN Device Transformerless PV Inverter 118 Design-Oriented Equivalent Circuit Model for Resonant Converters 108 Transmitter Coil Design for Free-Positioning Omnidirectional Wireless 119 Critical-Conduction-Mode-Based Soft-Switching Modulation for Three- Power Transfer System Phase PV Inverters with Reactive Power Transfer Capability 109 Shielding Study of a 6.78 MHz Omnidirectional Wireless Power Transfer 120 Improved Three-Phase Critical-Mode-Based Soft-Switching Modulation System Technique with Low Leakage Current for PV Inverter Application 110 The LCCL-LC Resonant Converter and Its Soft Switching Realization for 121 Balance Technique for CM Noise Reduction in Critical-Mode-Based Three- Omnidirectional Wireless Power Transfer Systems Phase Soft Switching Bidirectional AC-DC Converters 111 Three-Phase Interleaved LLC Resonant Converter with Integrated Planar 122 PCB Winding Coupled Inductor Design for Critical-Mode-Based Three- Magnetics Phase Bidirectional AC-DC Converters with Balance Technique 112 A High-Efficiency, High-Density, Wide-Bandgap, Device-Based 123 High Frequency Transformer Design with High-Voltage Insulation for Bidirectional On-Board Charger Modular Power Conversion from Medium-Voltage AC to 400 V DC 113 Optimal Design of Planar Magnetic Components for a Two-Stage, GaN-Based DC-DC Converter 104 NUGGETS | PMC Low Loss Integrated Inductor and Transformer Structure and Application in Regulated LLC Converter for 48 V Bus Converter ntermediate bus architectures employing 48 V bus converters are widely used in telecom power supply applications. With the rapid Iincrease of demanded power by these loads, higher efficiency and power density are driving for better performance power manage- ment solutions. In this work, a single stage LLC converter with integrated mag- netics is proposed for 48 V/12 V-1 kW bus converters that can pro- vide both regulation and isolation. The current practice for dc-dc bus converters is to operate at low switching frequencies, 100-200 KHz, with power density below 400 W/in3. For higher power density, this converter will operate at a 10-times higher frequency than indus- try practice. The LLC converter is most suitable for high-frequency operation due to its soft switching properties for all devices so that higher efficiency and power density can be realized. For high output current and low voltage dc-dc converters, the matrix converter has proven to be a perfect candidate as it distributes the secondary cur- rent among different outputs to reduce the total conduction loss. In this work, two matrix transformers, each with two trans- former outputs, are used to deliver a 1 kW output power. The two transformers are connected in parallel from both primary and sec- ondary windings as shown in Fig. 1; the primary windings are ar- ranged in a way to integrate the parallel transformers with one core Fig. 1. Single stage LLC DC/DC converter with matrix transformer. structure. To achieve output voltage regulation, the resonant inductor needs to be designed to a specific value to have the required regula- tion capabilities. A novel magnetic structure is proposed where the transformer primary windings are extended with the addition of a magnetic core to achieve a controllable leakage inductance to achieve the regulation capabilities. The shared winding will result in lower losses of the resonant inductor. The proposed PCB winding arrange- ment is shown in Fig. 2. A prototype has been developed for the pro- posed converter, achieving a power density of 800 W/in3 with an es- timated efficiency > 97% higher than all available industry practices. Fig. 2. Integrate matrix transformer and inductor PCB winding arrangements. CPES ANNUAL REPORT 2020 105 Magnetic Integration of Matrix Transformer with a Highly Controllable Leakage Inductance he 48 V power architecture is attracting significant attention for the datacenter industry. The 48 V power architecture pro- Tvides high efficiecncy and is more suitable for high-power delivery as opposed to the legacy 12 V bus architicture. The power rating per server rack in a datacenter is increasing significantly to 24 kW or higher. A number of parallel 3 kW power supply modules are used. This paper focuses on the design of a 400 V/48 V 3 kW dc-dc converter with high efficiency and power density. A full bridge CLL circuit is used with a matrix of four transformers. This paper proposes a novel approach to integrate high con- trollable leakage inductance with a matrix of four transformers us- ing one magnetic core. The paper proposes a five-leg magnetic core where four legs are utilized for four transformers and a fifth leg (cen- ter leg) is placed at the center between the four transformer legs to create controllable leakage inductance without sacrificing the power Fig. 1. Proposed 5-leg core with proposed winding arrangement. density of the magnetic structure, as shown in Fig. 1. The secondary windings of the original matrix transformers are relocated to create leakage flux in the center leg. Different winding arrangements can also be used to create different leakage inductances on the secondary or primary sides. The leakage inductance can be controlled by vary- ing the cross-section area and air gap of the center leg. In Fig. 2, the secondary windings are relocated without making any change on the primary windings. The blue secondary windings are active for the first half cycle, and red secondary windings are active for the second half cycle. This will cause leakage flux to pass through the center leg. By relocating the secondary winding, the physical leakage induc- tance is found on the secondary side. This way, the proposed wind- ing structure can be used in a three-element CLL resonant circuit, as shown in Fig. 2. Different combinations of winding arrangements will result in different leakage inductance values in both primary and secondary sides. In summary, the paper proposes a novel magnetic structure with 5-leg core. The magnetic structure integrates a matrix of four trans- formers with high controllable leakage inductance in a condensed footprint. The integrated magnetics can be applied to a 400 V/48 V 3 kW CLL resonant converter to implement a high-efficiency and high power density dc-dc converter for a datacenter power supply. Fig. 2. Full-bridge CLL circuit with matrix transformer and integrated inductor. 106 NUGGETS | PMC Control Technique for CRM-Based, High-Frequency, Soft-Switching Three-Phase Inverter Under Grid Fault Condition o develop a high power density and efficiency inverter for a the voltage sag case where the grid voltage and the output current are grid-tied application, a critical conduction mode (CRM)-based imbalanced, an undesired phenomenon results. As shown in Fig. 1, Tsoft-switching modulation (DPWM + CRM + DCM) for a continuous conduction mode (CCM) operation occurs where dis- three-phase, two-level inverter was developed. In this modula- continuous conduction mode (DCM) is intended. It should be noted tion scheme, one phase is clamped to a dc rail so that the other two that turn-on loss is mostly concentrated in the CCM region, creat- phases operate at high frequencies as CRM and DCM. Although it ing an extremely high likelihood that hardware might fail. The rea- was proven that the modulation method is a reasonable solution for son for CCM operation is due to the inherent characteristics of the high-frequency operation with high efficiency, previous studies only soft-switching modulation. The turn-on instant of the DCM phase focused on ideal grid conditions such as balanced and rated voltage. is synchronized with the CRM phase to reduce the wide switching- For practicality, studies on non-ideal grid conditions (like fault con- frequency range. Under voltage sag conditions, because of the de- ditions) need to be conducted. pendency of the DCM phase on the CRM phase, the V-sec. balance Voltage sag is one of the most frequent grid faults. Under this at the DCM phase is violated in a specific region. condition, the magnitude of 3-phase voltage decreases, and imbal- In order to prevent CCM operation and reduce the turn-on loss, ance appears between each phase, resulting in positive and negative pulse skipping is introduced in the DCM phase. By comparing zero sequence voltage. To avoid a large voltage ripple at the dc-link ca- current detection (ZCD) signals of the CRM phase and the DCM pacitor, which may lead to over-voltage protection and stop inverter phase, decision making for pulse skipping is made. In this control operation, constant active power should be delivered to the grid. For technique, the DCM phase current has enough time to reach zero. constant active power delivery, the output current to the grid be- Consequently, the concentrated turn-on loss in the CCM operation comes imbalanced. region diminishes significantly. However, if the soft-switching modulation is directly applied to Fig. 1. Operation mode of soft-switching inverter and turn-on Fig. 2. Control strategy under grid fault condition: pulse skipping in DCM phase. loss distribution under Type B voltage sag.
Details
-
File Typepdf
-
Upload Time-
-
Content LanguagesEnglish
-
Upload UserAnonymous/Not logged-in
-
File Pages21 Page
-
File Size-