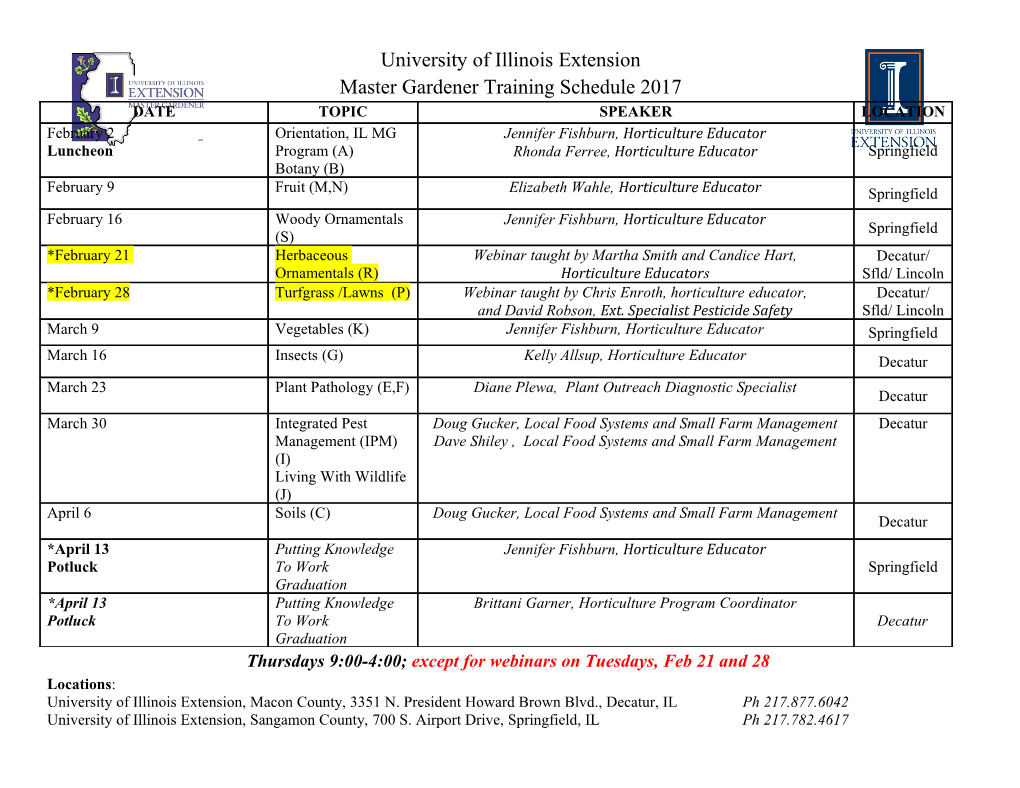
Downloaded from http://sp.lyellcollection.org/ by guest on September 30, 2021 The nature and tectonic significance of fault-zone weakening: an introduction E.H. RUTTER 1, R.E. HOLDSWORTH 2 & R.J. KNIPE 3 1Rock Deformation Laboratory, Earth Sciences Department, University of Manchester, Manchester M13 9PL, UK (e-mail: [email protected]) 2Reactivation Research Group, Department of Geological Sciences, University of Durham, Durham DH1 3LE, UK 3Rock Deformation Research, Earth Sciences Department, University of Leeds, Leeds LS2 9JT, UK Abstract: Fault zones control the location, architecture and evolution of a broad range of geological features, act as conduits for the focused migration of economically important fluids and, as most seismicity is associated with active faults, they also constitute one of the most important global geological hazards. In general, the repeated localization of dis- placements along faults and shear zones, often over very long time scales, strongly suggests that they are weak relative to their surrounding wall rocks. Geophysical obser- vations from plate boundary faults such as the San Andreas fault additionally suggest that this fault zone is weak in an absolute sense, although this remains a controversial issue. Our understanding of fault-zone structure and mechanical behaviour derive from three main sources of information: (1) studies of natural fault zones and their deformation pro- ducts (fault rocks); (2) seismological and neotectonic studies of currently active natural fault systems; (3) laboratory-based deformation experiments using rocks or rock-analogue materials. These provide us with a basic understanding of brittle faulting in the upper crust of the Earth where the stress state is limited by the frictional strength of networks of faults under the prevailing fluid-pressure conditions. Under the long-term loading con- ditions typical of geological fault zones, poorly understood phenomena such as subcritical crack growth in fracture process zones are likely to be of major importance in controlling both fault growth and strength. Grain-size reduction in highly strained fault rocks pro- duced in the plastic-viscous and deeper parts of frictional regime can lead to changes in deformation mechanisms and relative weakening that can account for the localization of deformation and repeated reactivation of crustal faults. Our understanding the interactions between deformation mechanisms, metamorphic processes and the flow of chemically active fluids is a key area for future study. An improved understanding of how fault- or shear-zone linkages, strength and microstructure evolve over large changes in finite strain will ultimately lead to the development of geologically more realistic numerical models of lithosphere deformation that incorporate displacements concentrated into narrow, weaker fault zones. In continental and oceanic regions, the defor- mining the location, modes of transport and mation of the Earth's crust (and lithosphere) is emplacement of economically important hydro- characteristically heterogeneous, with most dis- carbon reservoirs, hydrothermal mineral deposits placements being localized into linked systems and igneous intrusions. In addition, most active of faults and shear zones. In both intraplate and seismicity is associated with displacements plate margin settings, these approximately pla- along fault zones, which therefore represent one nar or tabular deformation zones influence of the most important global geological hazards. strongly the location, architecture and evolution of a broad range of geological features, includ- Fault-zone structure and ing rift basins, orogenic belts and transcurrent fault systems. Many fault zones are known to mechanical behaviour act as conduits for the focused migration of In the upper, seismogenic part of the crust, fluids and clearly play a central role in deter- deformation in fault zones occurs by frictional From: HOLDSWORTH,R.E., STRACHAN,R.A., MAGLOUGHLIN,J.F. & KNIPE, R.J. (eds) 2001. The Nature and Tectonic Significance of Fault Zone Weakening. Geological Society, London, Special Publications, 186, 1-11. 0305-8719/01/$15.00 9 The Geological Societyof London 2001. Downloaded from http://sp.lyellcollection.org/ by guest on September 30, 2021 2 RUTTER ET AL. I DEFORMATION I TYPICALFAULT I REGIME J ROCKS 2000 Earth's Surface 0 2 DISCRETE FAUL TS Breccia/gouge ITi ~" 1600 (Temperature-insensitive,j Catactasites 0 FRICTIONAL Fault googes 5 -- REGIME p.,...... e.,~j 6oooc Catactasitos, Depth crush melenges, (km) faultgo.ges [~ 1200 .............................(ca,,t.,ete~a~,~) 1~ 10 FRICTIONAL- PLASTIC/VISCOU; Semi.brittle mflonites TRANSITIONAL REGION Pseudotachylytes (Dep~ is Phyltonites ~: 800 - rock-type L5 15 -- - se_ns_i~).. .................... Myfonites /f/ IntracrystallinePlasticity PLASTIC/VISCOU~ if (tem~ratur~sensitive,pressur~insensitive) REGIME 400 900oC 20-- Blastomflonites lOOm , Fig. 2. A widely accepted conceptual model for the o 460 860 12'oo 1~o way that the character of a crustal fault zone might ConfiningPressure (MPa) vary with depth (based on Sibson 1977). Narrow, brittle-frictional faults with a range of possible cata- Fig. 1. Simplified representation of the data of Tullis clastic fault rock products pass with increasing depth & Yund (1977) for the ultimate strength of Westerly into foliated mylonitic fault rocks in which intracrys- granite at a strain rate of c. 10-Ss-1; frictional talline plastic and diffusion-accommodated viscous strength behaves in much the same way. The figure flow processes progresssively dominate. The transi- shows that strength in the brittle regime (up to tional region between the upper and lower flow 300 ~ is insensitive to temperature, but very sensi- regimes is expected to correspond to a crustal tive to confining pressure, giving way at higher tem- strength maximum. The horizontal scale length is peratures to an increased temperature sensitivity, but greatly exaggerated relative to the vertical. Although a reduction in pressure sensitivity as intracrystalline the fault zone is shown broadening with depth, this plastic processes begin to dominate. may not necessarily occur, depending on rock type. processes in which the deformation mechanisms and local conditions (slip velocity, effective involve brittle fracture and frictional sliding. pressure, temperature). Except in initially porous rocks, these processes At greater crustal depths and hence higher lead to dilatancy. Thus, the strength of brittle temperatures, brittle-frictional faults pass faults increases with effective pressure and downward into shear zones (e.g. Fig. 2) and the hence depth of burial (Fig. 1; Byeflee 1978; regime changes to one of viscous flow in which Paterson 1978; Sibson 1983). Recurrence of a range of non-frictional, thermally activated movements on localized faults usually points to deformation mechanisms are involved to pro- the fault zone being weaker than the stress duce crystal plasticity and diffusional creep required to form a fresh fault in the surrounding (Sibson 1977; Tullis & Yund 1977; Schmid & protolith. This degree of weakening after fault Handy 1991). In the region separating these two initiation is probably due to some combination regimes, a frictional-viscous (or sometimes of the formation of fragmentary rock products called brittle-ductile) transition is likely to that are more porous and less cohesive than the coincide with a strength maximum in the litho- protolith (thereby allowing enhanced fluid-rock sphere, based on the findings of laboratory interaction) coupled with the development of a experiments and seismological studies (e.g. foliated fabric, which may involve local concen- Sibson 1977, Sibson, 1983). If the deformation tration of clay minerals. Whether the fault becomes isovolumetric, the stable continuation motion is steady or seismogenic depends on of localized flow demands that the material whether the fault zones display transiently vel- inside the fault zone be weaker than that out- ocity-strengthening or -weakening character- side. It is clear, however, that even high-tem- istics (Scholz 1990, Scholz, 1998). This perature shearing can be accompanied by some characteristic is sensitive both to fault rock type dilatancy, which may be crucial to explain the Downloaded from http://sp.lyellcollection.org/ by guest on September 30, 2021 FAULT ZONE WEAKENING 3 ability of deep shear zones to transport fluids, sources of information: (1) studies of natural both aqueous and melts (Bruhn et al. 2000). fault zones and their deformation products (fault Even at the very high pressures in the deeper rocks); (2) seismological and neotectonic studies parts of subduction zones (400-600km depth), of currently active natural fault systems; (3) lab- localized deformation is indicated by the occur- oratory-based deformation experiments using rence of earthquakes whose first-motion pat- rocks or rock-analogue materials. Natural fault terns indicate shear faulting. These too demand zones generally preserve fault rocks whose com- a dramatic weakening process that is either iso- position and microstmcture can be used to gain volumetric or compactive in nature, so that insights into the nature and evolution of defor- there is no requirement for work to be done mation mechanisms and the theological beha- against the enormous effective pressures at viour of fault zones under a wide range of such depths (Kirby et al. 1996). pressure and temperature conditions (e.g. Handy The repeated localization
Details
-
File Typepdf
-
Upload Time-
-
Content LanguagesEnglish
-
Upload UserAnonymous/Not logged-in
-
File Pages11 Page
-
File Size-