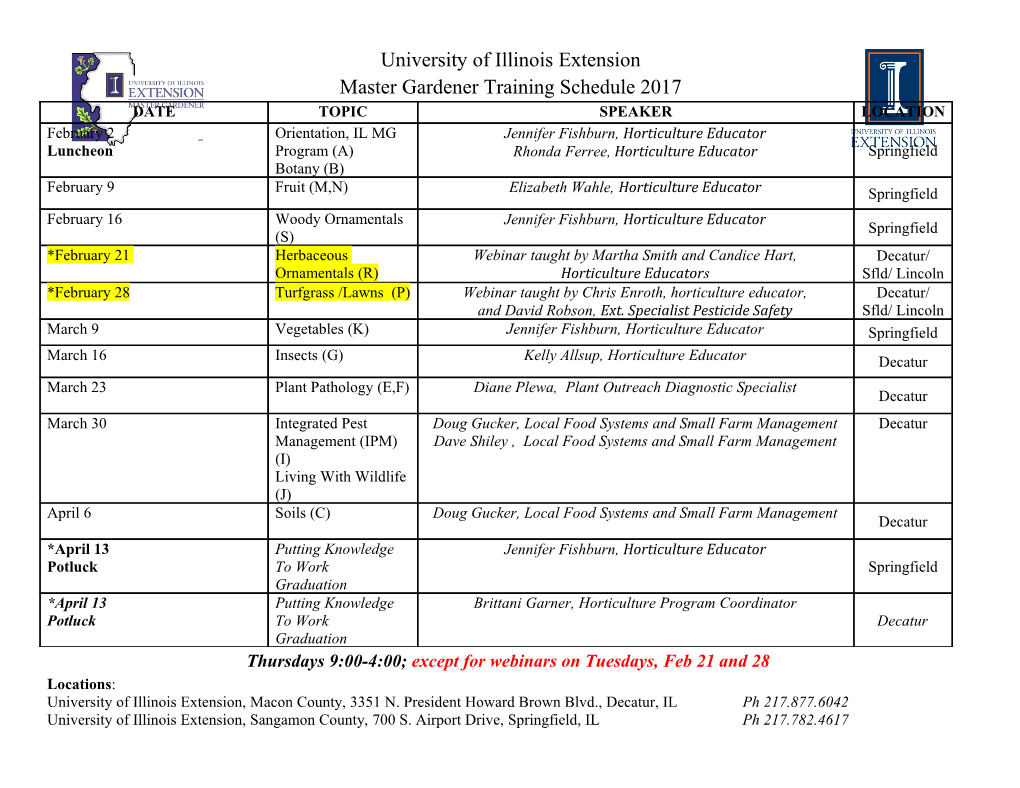
INSULIN-LIKE GROWTH FACTOR SIGNALING DURING MYOGENESIS by Hongxia Ren A dissertation submitted in partial fulfillment of the requirement for the degree of Doctor of Philosophy (Molecular, Cellular and Developmental Biology) in The University of Michigan 2009 Doctoral Committee: Professor Cunming Duan, Chair Professor Christin Carter-Su Professor Robert J. Denver Professor Daniel J. Klionsky ACKNOWLEDGEMENTS I have learned and experienced a lot over the years in Ann Arbor. Had it not been the generous help and warm encouragement from the people around me, I probably wouldn’t have come this far. I feel grateful beyond measure to my mentor Dr. Cunming Duan and, I must say, to the fates that allowed my path to cross his. Dr. Duan has taught me numerous things in scientific research and teaching. To this day, I am still learning from him. His integrity, generosity, intense devotion, and perseverance are what inspired me most. And, for their extraordinary grace and kindness, I offer my thanks to Dr. Duan’s family, who helped me through my most difficult times. I am grateful to my committee members – Dr. Carter-Su, Dr. Denver, and Dr. Klionsky for their guidance over the years. Dr. Carter-Su kindly shares her expertise in growth factor research. Her insightful suggestions and attention to details have always been critical inputs. Dr. Denver’s generous help is an invaluable part of my graduate school training. Dr. Klionsky’s dedication has been and will always be an inspiration for me. I want to express my gratitude to members in Duan lab, Denver lab, and Cadigan lab, past and present, for supporting the projects, encouraging me, and lending painstaking assistance. I wish to offer special thanks to Dr. Ping Yin and Dr. Peter Schlueter. Finally, I would like to acknowledge my family and friends for their unconditional love and support. Most importantly, I thank my parents for supporting me financially, physically, and spiritually. ii TABLE OF CONTENTS ACKNOWLEDGEMENTS ii LIST OF FIGURES vi CHAPTER I: INTRODUCTION 1 Myogenesis 1 Muscle and myogenesis 1 Transcription factors in myogenesis 3 Signaling pathways regulating myogenesis 6 IGF 8 The IGF ligands 8 The IGF-I receptor 11 The IGF-II receptor 14 The IGF binding proteins 15 The function of IGFBPs 19 Cellular actions of IGFBPs 19 In vivo functions of IGFBPs 21 iii Post-translational regulation of IGFBPs 24 IGF and muscle 26 IGF and muscle biology 26 IGFBP-5 and myogenesis 28 Project summary 31 CHAPTER II: IGFBP-5 REGULATES MUSCLE CELL DIFFERENTIATION BY BINDING TO IGF-II AND SWITCHING ON THE IGF-II AUTO-REGULATION LOOP 38 Abstract 38 Introduction 39 Results 42 Discussion 52 Material and Methods 57 Acknowledgements 62 Chapter III: HYPOXIA CONVERTS THE MYOGENIC ACTION OF IGF INTO MITOGENIC ACTION BY REPROGRAMMING GLUCOSE METABOLISM AND MULTIPLE SIGNALING PATHWAYS 80 Abstract 80 Introduction 81 Results 83 iv Discussion 95 Material and Methods 102 Acknowledgements 107 CHAPTER IV: CONCLUSIONS AND FUTURE DIRECTIONS 128 IGFBP-5 potentiates IGF actions during myogenesis 128 Hypoxia converts IGF actions by reprogramming its signaling cascades and cellular metabolism 134 BIBLIOGRAPHY 151 v LIST OF FIGURES Figure 1.1 Major transcription factor network in myogenesis 34 Figure 1.2. Illustration of major signaling pathways regulating myogenesis 35 Figure 1.3. IGF-IR and major downstream effectors in IGF signaling pathway 36 Figure 1.4. Schematic representation of IGFBP-5 domain arrangement 37 Figure 2.1 The induction of IGFBP-5 precedes that of IGF-II during myogenesis 63 Figure 2.2. Knockdown of IGFBP-5 impairs myogenic differentiation 64 Figure 2.3. Knockdown of IGFBP-5 decreases Myogenin expression 65 Figure 2.4. Forced expression of Myogenin does not rescue the myogenic defects in IGFBP-5 deficient cells 66 Figure 2.5. Knockdown of IGFBP-5 suppresses IGF-II gene expression and decreases IGF-IR-mediated signaling activity 68 Figure 2.6. Exogenous IGFs or expression of a constitutively active Akt “rescues” the myogenic defects caused by IGFBP-5 knockdown 70 Figure 2.7. IGF-II up-regulates its own gene expression through the PI3K-Akt signaling pathway 72 Figure 2.8. IGFBP-5 does not exert direct effect on IGF-II gene expression 74 Figure 2.9. IGFBP-5 is localized on the cell surface and promotes myogenic differentiation by binding to and promoting IGF-II action 76 Figure 2.10. The IGF-II and IGFBP-5 genes exhibit similar induction patterns and IGFBP-5 knockdown inhibits myogenic differentiation in primary skeletal muscle cells 78 vi Figure 3.1. IGF stimulates differentiation under normoxic conditions but promotes proliferation under hypoxic conditions through the activation of the same receptor 108 Figure 3.2. Hypoxia converts the myogenic action of IGF into mitogenic action through HIF-1-dependent mechanisms 112 Figure 3.3. Hypoxia reprograms glucose metabolism and this action is enhanced by IGF stimulation 114 Figure 3.4. Hypoxia represses mTOR signaling during myogenesis and the inhibition of mTOR activity abolishes the myogenic action but not the mitogenic action of IGF 116 Figure 3.5. Hypoxia represses Akt signaling activity, and expression of constitutively active Akt restores the mTOR activity and myogenic action of IGF under hypoxic conditions 118 Figure 3.6. Hypoxia alters IGF actions by differentially regulating Erk1/2 and p38 MAPK pathways 122 Figure 3.7. Schematic diagram illustrates the mechanisms by which hypoxia alter the myogenic and mitogenic actions of IGF during myogenesis 126 Figure 4.1. IGF-I and IGF-II transcription is differentially regulated by IGF-II stimulation during myoblast differentiation 149 Figure 4.2. IGFBP-5 siRNA decreases cell number with a concomitant increase in apoptosis in at early phase of differentiation 150 vii CHAPTER I INTRODUCTION Myogenesis Muscle and myogenesis In mammals, muscle constitutes a large part of the body mass. Muscle is a tissue specialized for contraction. Based on the difference in function, structure, and development, muscle tissue can be divided into four main categories – skeletal muscle, cardiac muscle, smooth muscle, and myoepithelium. This thesis will focus on the molecular mechanisms regarding skeletal muscle development. Skeletal muscle, under the control of nervous system, is responsible for voluntary movements. Mammalian skeletal muscle is composed of large cells with elongated shape. Therefore, these muscle cells are often referred to as muscle fibers. There are two broad types of voluntary muscle fibers: slow twitch and fast twitch, which are innervated by different motor neurons (Pette, 2002). Slow twitch fibers contract for long periods of time but generate less force, while fast twitch fibers contract more rapidly and powerfully but fatigue very rapidly. The different contractile properties are imparted by the expression of specific myosin isoforms. Recent studies have revealed that fiber composition is regulated by signaling molecules and transcriptional factors, such as calcineurin, CaM kinase, Hedgehog, and peroxisome-proliferator-activated receptor-gamma co-activator (PGC) (Arany et al., 2007; Blagden et al., 1997; Du et al., 1997; Lin et al., 2002). 1 Each skeletal muscle fiber is a syncytium, containing many nuclei within a common cytoplasm. The multinucleated skeletal muscle cells are formed by the fusion of muscle precursor cells – myoblasts. Myogenesis is a highly ordered process (Buckingham et al., 2003). During embryonic development, the muscle precursor cells commit to myogenic linage. After a period of proliferation, the myoblasts withdraw from cell cycle, express muscle-specific genes, and eventually fuse with one another to form multinucleated myotubes. This process is known as myoblast differentiation. It is subjected to the intricate regulation of intrinsic muscle specific transcription factors, such as myogenic regulatory factors (MRFs) (Weintraub, 1993), and extrinsic growth hormones. Mature muscle cells express muscle-specific proteins matching with specific physical activities. Of note, before myoblast differentiation, the presence of these proteins is either not detectable or exist in very low concentrations. Proteins, that are parts of the contractile apparatus, are abundantly expressed, including specific isoforms of actin, myosin, tropomyosin, and troponin. Muscle tissue consumes much of the body’s energy and produces adenosine triphosphate (ATP) to power the contractile apparatus. In line with this, muscles adopt specialized metabolism by expressing creatine kinase to regenerate ATP during burst activity (van Deursen et al., 1993). Mature muscle cells also express acetylcholine receptors on the surface within specialized neural muscular junction called synapse, which renders them sensitive to the stimuli coming from the nerve terminal. Terminal myoblast differentiation and proliferation are often considered as two mutually exclusive events. After differentiation, the multinucleated muscle cells do not divide and the nuclei stop replicating their DNA. In humans, the number of multinucleated skeletal muscle fibers is determined before birth. Postnatal muscle growth is mainly achieved 2 by muscle hypertrophy – the increase in the size of muscle fibers without an increase in muscle fiber number. The increase in muscle fiber size is achieved by individual myoblasts being recruited to and subsequently fusing with existing multinucleated muscle fibers. Transcription factors in myogenesis Myoblast differentiation is controlled by at least two families of transcription factiors – the MyoD family
Details
-
File Typepdf
-
Upload Time-
-
Content LanguagesEnglish
-
Upload UserAnonymous/Not logged-in
-
File Pages192 Page
-
File Size-