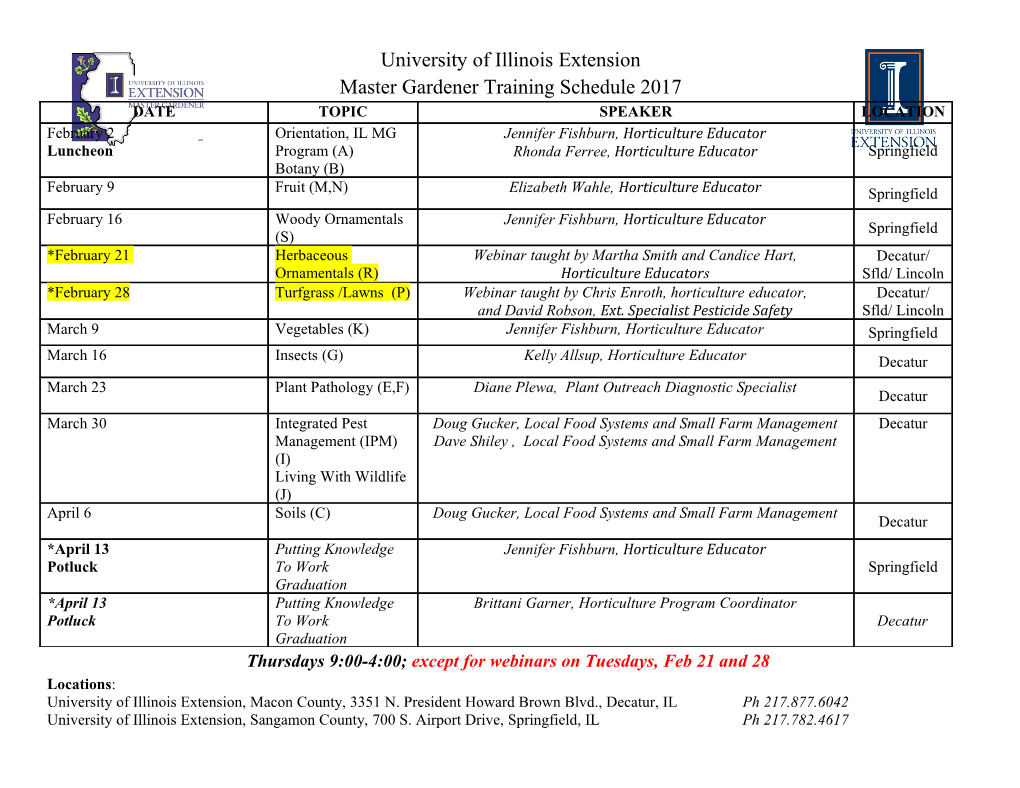
Vol. 520: 35–56, 2015 MARINE ECOLOGY PROGRESS SERIES Published February 3 doi: 10.3354/meps11116 Mar Ecol Prog Ser FREEREE ACCESSCCESS Phytoplankton morphology controls on marine snow sinking velocity Emmanuel C. Laurenceau-Cornec1,2,3,*, Thomas W. Trull2,3, Diana M. Davies2, Christina L. De La Rocha4, Stéphane Blain5,6 1Institute for Marine and Antarctic Studies, University of Tasmania, Private Bag 129, Hobart, Tasmania 7001, Australia 2Antarctic Climate and Ecosystems Cooperative Research Centre, University of Tasmania, Private Bag 80, Hobart, Tasmania 7001, Australia 3Commonwealth Scientific and Industrial Research Organisation, Marine and Atmospheric Research, Castray Esplanade, Hobart, Tasmania 7000, Australia 4CNRS, UMR 6539, Institut Universitaire Européen de la Mer, Université de Bretagne Occidentale, Technopôle Brest-Iroise, Rue Dumont d’Urville, Plouzané 29280, France 5CNRS and 6UPMC Université Paris 06, UMR 7621, LOMIC, Observatoire Océanologique, Banyuls-sur-Mer 66650, France ABSTRACT: During the second KErguelen Ocean and Plateau compared Study (KEOPS2) in October−November 2011, marine snow was formed in roller tanks by physical aggregation of phytoplankton assemblages sampled at 6 stations over and downstream of the Kerguelen Plateau. Sinking velocities, morphology, bulk composition (transparent exopolymer particles, biogenic sil- ica, particulate organic carbon), and phytoplankton contents were measured individually on 66 aggregates to identify controls on sinking velocities. Equivalent spherical diameters (ESD) ranged from 1 to 12 mm, and the particle aspect ratios, Corey shape factors, and fractal dimensions (DF1 = 1.5, DF2 = 1.8) were close to those of smaller natural aggregates (0.2 to 1.5 mm) collected in poly- acrylamide gel-filled sediment traps (DF1 = 1.2, DF2 = 1.9). Sinking velocities ranged between 13 and 260 m d−1, and were correlated with aggregate size only when considering individually the experiments conducted at each station, suggesting that a site-dependent control prevailed over the general influence of size. Variation in dominant diatom morphologies among the sites (classi- fied as small spine-forming or chain without spines) appeared to be a determinant parameter influencing the sinking velocity (SV [m d−1] = 168 − 1.48 × (% small spine-forming cells), r 2 = 0.98), possibly via a control on species-specific coagulation efficiency affecting particle structure and excess density. Our results emphasize the importance of ecological considerations over that of simple compositional perspectives in the control of particle formation, and in accurate parameter- izations of marine snow sinking velocities that are essential to predictions of biological carbon sequestration. KEY WORDS: Sinking velocity · Marine snow · Aggregation · Phytoplankton · Porosity Resale or republication not permitted without written consent of the publisher INTRODUCTION dioxide, and has become known as the ‘oceanic bio- logical carbon pump’ (Volk & Hoffert 1985). Substan- In the ocean, the rain of large biogenic particles is tial production of particulate organic matter (POM) in a major pathway for the export of carbon from the the euphotic zone is essential but not sufficient to surface to the interior (Suess 1980, Fowler & Knauer obtain high carbon export (Buesseler 1998). The 1986, Asper et al. 1992). This mechanism contributes speed at which particles settle is also a critical para - significantly to the drawdown of atmospheric carbon meter since it determines the duration of their expo- *Corresponding author: [email protected] © Inter-Research 2015 · www.int-res.com 36 Mar Ecol Prog Ser 520: 35–56, 2015 sure to extensive physical and biological transforma- parameters, the shape (McNown & Malaika 1950, tions (Karl et al. 1988, Boyd et al. 1999) responsible Alldredge & Got schalk 1988), porosity or compact- for the observed attenuation of the particle flux with ness (Kajihara 1971, Iversen & Ploug 2010), perme- depth (Martin et al. 1987, Silver & Gowing 1991). ability (Matsumoto & Suganuma 1977), fractal struc- In the Southern Ocean, a region known to play a ture (Johnson et al. 1996, Engel et al. 2009), content leading role in global climate and carbon systems of high density minerals (Armstrong et al. 2001, (Sigman & Boyle 2000), primary productivity is low Klaas & Archer 2002, Passow & De La Rocha 2006) due to phytoplankton growth restriction by a combi- or low density particulate polysaccharides (Engel & nation of iron and light limitation (Martin 1990, de Schartau 1999, Azetsu-Scott & Passow 2004), phyto- Baar et al. 1995). These waters are described as plankton species (Passow 1991, Padisák et al. 2003) ‘high nutrient, low chlorophyll’ (HNLC), and contain and their physiological state (Bienfang et al. 1982, a large amount of unused surface macro nutrients Waite et al. 1992, Muggli et al. 1996) or individual capable of fuelling the production of high quantities organism size (Boyd & Newton 1995, Waite et al. of organic carbon and potentially its se questration. 1997a) have been proposed as potential controls of Against this background, like oases in deserts, some particle sinking velocity. Due to this high complexity areas in the Southern Ocean are fertilized by a and the absence of clear controlling factors provided natural supply of iron and display remarkable sea- by empirical research, most biogeochemical models sonal biomass increases (Sullivan et al. 1993), offer- have no other alternative than simple sinking velocity ing a great opportunity to investigate the controls parameterization (e.g. constant or size-dependent). on carbon export efficiencies in the context of iron Several modelling studies have focused on the sen- fertilization. sitivity to sinking velocity para meterization, testify- This study focuses on the bloom that occurs each ing to the awareness that improvements are needed year between October and February over and down- and that more complexity needs to be added to the stream of the Kerguelen Plateau in the Indian sector models (Gehlen et al. 2006, Kriest & Oschlies 2008, of the Southern Ocean (Blain et al. 2007). In Janu- 2013, Losch et al. 2014). Here, we investigate a ary−February 2005, the first KErguelen Ocean and comprehensive set of morphological and composi- Plateau compared Study (KEOPS1) demonstrated tional parameters in a single study to identify poten- that the downward flux of carbon was elevated by a tial influences on particle sinking velo city and to de - factor of 2 to 3 in comparison to the surrounding velop a possible explanation for the in consistencies HNLC waters (Savoye et al. 2008). Particle size spec- often observed between sinking velo city and parti- tra from an in situ camera demonstrated that more cle size, offering a potential alternative for biogeo- and larger particles were present beneath the bloom chemical model parameterization. (to 400 m depth) than in surrounding HNLC waters, although a comparison to size spectra of particles col- lected in gel-filled sediment traps showed slower Theory sinking velocities (Jouandet et al. 2011), suggesting that sinking velocity was not a simple monotonic We refer the reader to the classic textbook on fluid function of particle size. dynamics from Batchelor (1967) for further details on According to theory and empirical studies, size is the theory of particle sinking velocity. known to exert a major control on particle sinking For a sphere settling in a fluid, the immersed velocity (Dietrich 1982, Gibbs 1985), but discrepan- weight (Wi) is the difference between the force of cies in the size−sinking velocity relationship are gravity acting downward and the buoyancy force often reported, and other parameters (e.g. particle acting upward, and is given by (e.g. Komar & composition or structure) can prevail over size in the Reimers 1978): control of the sinking velocity, as previously noted π (Diercks & Asper 1997, Engel & Schartau 1999, WD= 3()ρ−ρ g (1) i 6 sf Iversen & Ploug 2010). While extensive study of these parameters has verified their importance, it where D is the diameter of the sphere, ρs and ρf are has also turned out to be difficult to define a limited the sphere and fluid densities, respectively, and g number of parameters suitable to predict carbon (9.81 m s−2) is the acceleration due to gravity. The export accurately — something that would be of Reynolds number (Re; Reynolds 1883) is the para - considerable use in computationally expensive mod- meter that determines a flow field for boundaries of a els of ocean biogeochemical cycling. Among these given form and is given by: Laurenceau-Cornec et al.: Phytoplankton controls on sinking velocity 37 UD by Stokes, Eqs. (5) & (6) are only valid at small Re = s (2) ν Reynolds number, empirically found to apply to Re where Us is the sphere sinking velocity and ν is the < 0.5 (Graf & Acaroglu 1966, White 1974, Komar & kinematic viscosity of the fluid. Reimers 1978). Particles termed ‘marine snow’ are large aggre- By comparing the general model (Eq. 4) to its par- gates (>0.5 mm) formed by physical aggregation of ticular case represented by the Stokes’ expression phytoplankton and miscellaneous debris: mostly (Eq. 6), one can notice that the settling velocity has a fecal pellets, inorganic particles and zooplankton weaker dependence on size in the general model feeding structures (Alldredge & Silver 1988, All- since the diameter is raised to the power of 0.5, rather dredge & Gotschalk 1990). Because of their large size than 2 as in the Stokes’ law. and
Details
-
File Typepdf
-
Upload Time-
-
Content LanguagesEnglish
-
Upload UserAnonymous/Not logged-in
-
File Pages22 Page
-
File Size-