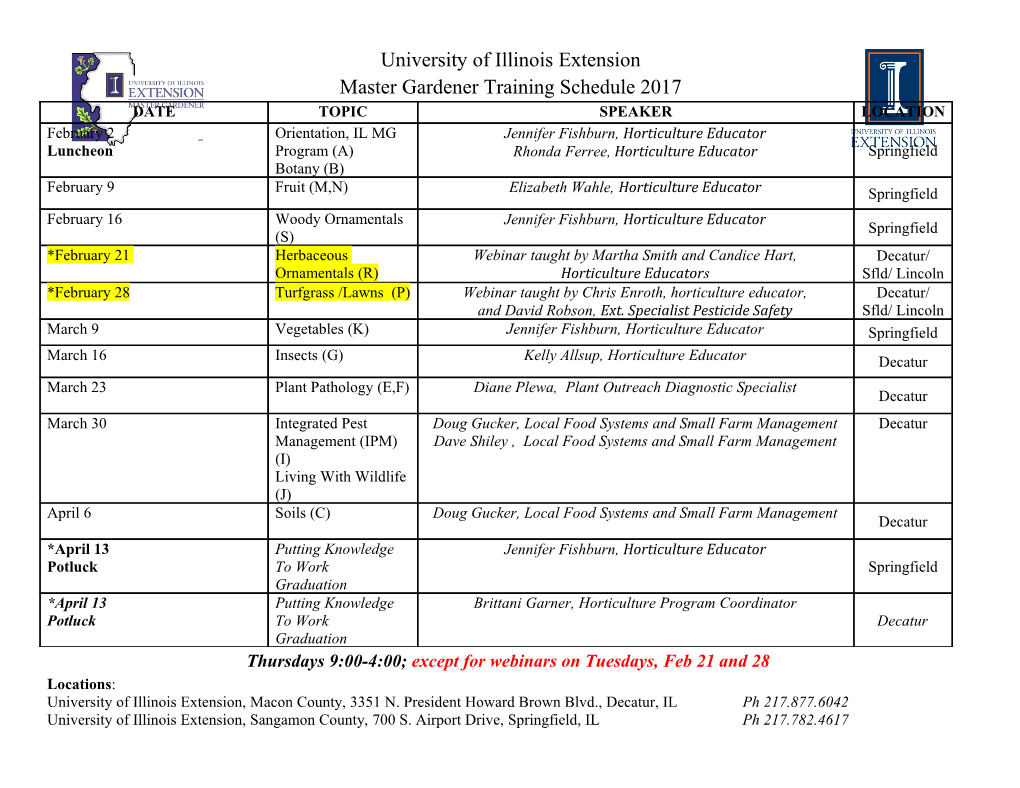
Title/Name of the area: NAA Presented by Maria Ana Dionísio (PhD in marine sciences), with a grant funded by Instituto da Conservação da Natureza e das Florestas and ISPA - Instituto Universitário de Ciências Psicológicas, Sociais e da Vida, [email protected] Pedro Ivo Arriegas, Instituto da Conservação da Natureza e das Florestas, [email protected] Abstract NAA (North of the Azores Area) EBSA is compounded by a total of 7 seamounts and one hydrothermal vent, this area includes one OSPAR high-seas Marine Protected Area - Mid Atlantic Ridge North of Azores (MARNA). The structures described and included are hotspots of marine life and in general they represent areas of an enhanced productivity, especially when compared with nearby abyssal areas. The Moytirra is the first known deep-sea hydrothermal vent field on the slow- spreading Mid-Atlantic Ridge North of the Azores, giving a high level of uniqueness to the NAA. This EBSA has a total area of 634515 km2 with identified structures depths ranging from 660m (top of Sedlo seamount) to 3200m (bottom of Lukin-Lebedev seamount). The area presents particular features which make this area eligible as an EBSA when assessed against the EBSA scientific criteria. All structures included in the NAA EBSA fulfill four or more out of the seven EBSA scientific criteria. The Sedlo bank is recently and extensively studied. A total of 536 species are present in this EBSA of which 6% are protected under international or regional law. The EBSA area is totally located under Portuguese national jurisdiction, with 7 of the 8 structures located on the extended continental shelf (seabed) and 1 (Sedlo) on the Portuguese EEZ close to Azores. Introduction The NAA EBSA includes a total of 7 seamounts (Altair, Antialtair, Chaucer, Cherkis, Crumb, Lukin- Lebedev and Sedlo), 1 hydrothermal vent (Moytirra) and the OSPAR high-seas Marine Protected Area (MARNA). These structures present particular features which make this area eligible as an EBSA when assessed against the EBSA scientific criteria. The majority of the EBSA structures (7) are classified as a seamount structure. The seamounts are defined as isolated topographic features of the seabed that have a limited lateral extent and rise more than 1000 m from abyssal depths (Menard, 1964). Large seamounts are usually originate as volcanoes and primarily associated with the intraplate hotspots and mid-ocean ridges (Staudigel et al., 2010). Generally, seamounts topography may act as an element which turns the structures into high complexity sites. Due to their more or less isolated location, these structures can be an obstacle to the free circulation of the oceans. This gives rise to different kinds of phenomena and disturbances, including an increase in the speed of sea currents, upwellings, turbulence, Taylor cones, eddies, and NAA 1 even jets in the zones where the seamounts interact with ocean currents (Richardson et al., 2000; Kunze & Smith, 2004; White et al., 2007; Pakhorukov, 2008). Seamounts are hotspots of marine life (e.g. Rogers, 1994; Gubbay, 2003; Morato & Pauly, 2004; Pitcher et al., 2007, 2010; Mendonça et al., 2012), and in general represent areas of enhanced productivity in comparison with nearby abyssal areas. In most cases, around the seamounts there is an extensive anticyclonic eddy associated with the lifting of nutrients from the rich deep water, giving rise to high concentrations of nitrates and chlorophyll in shallow waters (Coelho & Santos, 2003), which encourages the development of a wealth of flora and fauna on the structures, leading to exposed hard substrates and improved food conditions for epibenthic suspension feeders (e.g. Cartes et al., 2007 a), b); Genin & Dower, 2007) such as cold water corals or deep water sponges (e.g. Samadi et al., 2007; Sánchez et al., 2008), tunas (e.g. Yasui, 1986; Morato et al., 2010, Ressurreição & Giacomello, 2013), marine mammals (e.g. Cañadas et al., 2002; Correia et al., 2015), and other organisms which apparently feed on prey aggregations (e.g. Boehler & Sasaki, 1988; Porteiro & Sutton, 2007; Tabachnick & Menchenina, 2007). Seamounts are biologically distinctive habitats of the open ocean exhibiting a number of unique features (Rogers, 1994; Probert, 1999; Morato & Clark, 2007). These structures can host very distinctive biological communities that are different to the communities on nearby soft sediment dominated abyssal plain, and these particular places may attract pelagic fish including larger, commercially valuable vertebrate species (Beryx splendens) and Invertebrate (Charonia lampas) and other marine species like a top predators such as the shark (Prionace glauca), and reptile species as a loggerhead sea turtles (Caretta caretta) and protected marine mammals (Balaenoptera borealis) (see e.g. Holland & Grubbs, 2007, Kaschner, 2007, Santos et al., 2007). Benthic biological communities on seamounts are highly vulnerable to human activities. Many benthic species are long-lived and slow-growing, and not resilient to human impacts (Convention on Biological Diversity, 2008). Seamounts are locations for a broad range of current-topography interactions and biophysical coupling, with implications for both phyto and zooplankton. Seamounts appear to support relatively large planktonic and higher consumer biomass when compared to surrounding ocean waters, particularly in oligotrophic oceans. It has been a widely held view that in situ enhancement of primary production fuels this phenomenon, but this has recently been challenged (Genin & Dower, 2007). Productivity in oceanic settings depends on light and nutrient availability, while overall production is the result of productivity and accumulation of the phytoplankton. At a seamount, either a seamount- generated, vertical nutrient flux has to be shallow enough to reach the euphotic zone and the ensuing productivity retained over the seamount long enough to allow transfer to higher trophic levels, or the seamount must rely on allochthonous inputs of organic material to provide a trophic subsidy to resident populations (Clark et al., 2010). NAA 2 The EBSA area shelters the only hydrothermal vent known to date in the North of the Azores. Since a great part of the seamounts and other structures in these EBSA remains unexplored, the presence of more vent communities and more seamounts cannot be excluded with full confidence. Hydrothermal vent fields are a peculiar structure site where high temperature, mineral-rich fluids discharge from the seafloor following the circulation, heating, and geochemical reactions of seawater in oceanic crust (Corliss et al., 1979). Hydrothermal vents occur along spreading ridges (such as the mid-Atlantic ridge), fracture zones and back-arc basins (Gage & Tyler, 1991). They are produced by the intrusion of seawater in the upper levels of the crust through channels formed in cooling lava flows, reacting chemically with hot basalt inside the crust generating a reflux with then rising back to the sea-bed to vent as superheated water enriched with compounds such as sulphides, metals, CO2 and methane (Gubbay, 2002). These are particular locations where high temperature, mineral-rich fluids discharge from the seafloor following the circulation, heating, and geochemical reactions of seawater in oceanic crust (Corliss et al., 1979). Generally, hydrothermal vent fields cover small areas of the seabed. The vents can have a wide spectrum of distribution, ranging from the deeper Beebe Hydrothermal Vent Field, laying in the Cayman Trough, a deep section of the Caribbean south of Cuba at ~5000 meters (Tarasov et al., 2005; Connelly et al., 2012) to the shallower in the D. João de Castro Bank near to Azores at 20m depth (Cardigos et al., 2005; Tarasov et al., 2005). The hydrothermal vent fields play a primordial role sustaining abundant populations of faunal species in the deep sea by the autochthonous primary production of high chemosynthetic primary production (e.g. Lutz & Kennish, 1993). The process use the reduced compounds (typically hydrogen sulfide, methane, or hydrogen) in vent fluids to fix inorganic carbon (Karl et al., 1980) that can be oxidized by microbes to release energy for the formation of organic carbon from carbon dioxide, carbon monoxide, or methane (Van Dover et al., 2002). The chemosynthetic organisms may be present in the water column, at the seafloor as microbial mats, within sediments, fractures of crustal rocks or the sub- seabed, or/and in symbioses with larger multi-cellular organisms (Dubilier et al., 2008). This microbiota mediate the transformation of chemical energy, thereby facilitating the development and maintenance of densely populated ecosystems in which both biomass and faunal abundances are very much greater than is typical at the deep seafloor (e.g. Lutz & Kennish, 1993; Smith et al., 2008). The hydrothermal community sustains abundant populations in the deep sea by the autochthonous primary production of chemosynthetic microbes (Grassle, 1985). More than 400 new faunal species have been described from deep-sea hydrothermal vents (Desbruyeres et al., 2006), enhancing our knowledge of marine biodiversity (Van Dover et al., 2002). The NAA EBSA area (Figure 1) includes 8 different structures that fulfill at least four EBSA Criteria. There are differences in the information available for each structure included in the EBSA area (Table 1). The Sedlo seamount is one of the “most” explored in the EBSA area. The seamount started to be studied in 2002, and from these data until 2005, Sedlo was
Details
-
File Typepdf
-
Upload Time-
-
Content LanguagesEnglish
-
Upload UserAnonymous/Not logged-in
-
File Pages39 Page
-
File Size-