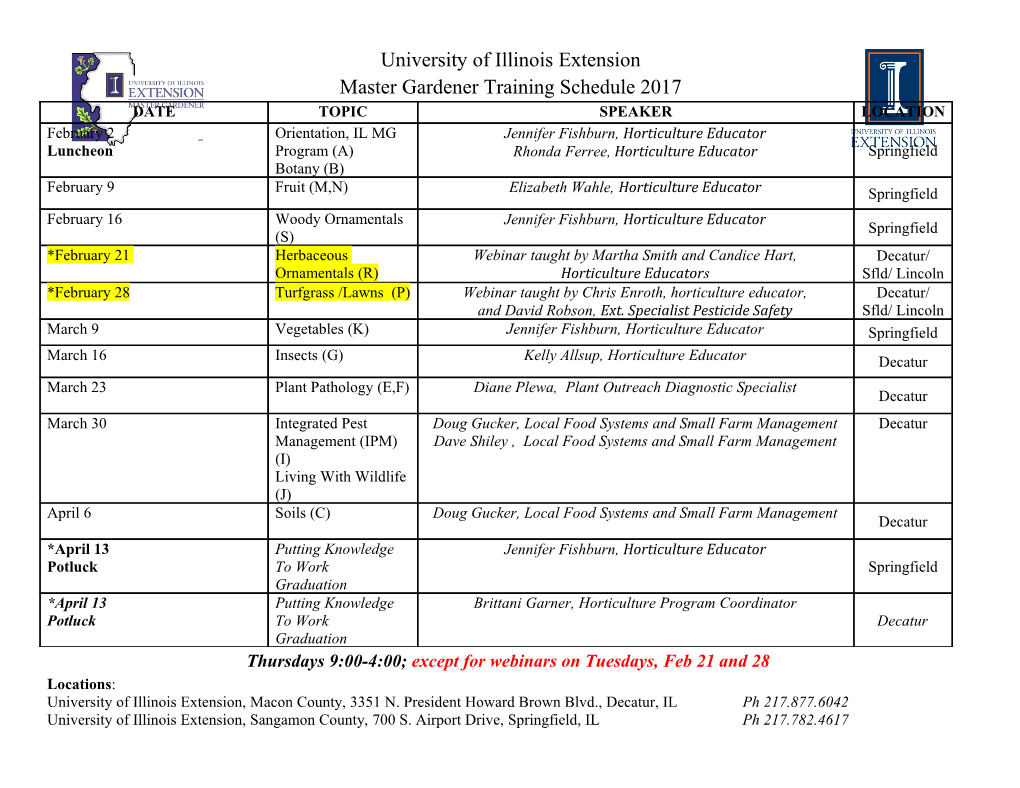
catalysts Article Biosynthesis of Medium- to Long-Chain α,!-Diols from Free Fatty Acids Using CYP153A Monooxygenase, Carboxylic Acid Reductase, and E. coli Endogenous Aldehyde Reductases Md Murshidul Ahsan 1 ID , Sihyong Sung 2, Hyunwoo Jeon 2, Mahesh D. Patil 2, Taeowan Chung 1 and Hyungdon Yun 2,* 1 School of Biotechnology, Yeungnam University, Gyeongsan 38541, Korea; [email protected] (M.M.A.); [email protected] (T.C.) 2 Department of Systems Biotechnology, Konkuk University, Seoul 050-29, Korea; [email protected] (S.S.); [email protected] (H.J.); [email protected] (M.D.P.) * Correspondence: [email protected]; Tel.: +82-2-450-0496; Fax: +82-2-450-0686 Received: 27 November 2017; Accepted: 22 December 2017; Published: 24 December 2017 Abstract: α,!-Diols are important monomers widely used for the production of polyesters and polyurethanes. Here, biosynthesis of α,!-diols (C8–C16) from renewable free fatty acids using CYP153A monooxygenase, carboxylic acid reductase, and E. coli endogenous aldehyde reductases is reported. The highest yield of α,!-diol was achieved for the production of 1,12-dodecanediol. In the nicotinamide adenine dinucleotide phosphate (NADPH) cofactor regeneration system, 5 g/L of 1,12-dodecanediol was synthesized in 24 h reaction from the commercial !-hydroxy dodecanoic acid. Finally, 1.4 g/L 1,12-dodecanediol was produced in a consecutive approach from dodecanoic acids. The results of this study demonstrated the scope of the potential development of bioprocesses to substitute the petroleum-based products in the polymer industry. Keywords: carboxylic acid reductase; CYP153A monooxygenase; diols; polyester; polyurethanes 1. Introduction The utilization of renewable feedstock-derived raw materials and development of bioprocesses thereof to substitute petroleum-based products in polymer industry has shown a steep surge in recent years [1–3] due to concern of future shortage of petroleum resources and in consideration of green production [4,5]. Compared to chemical catalysis, biocatalysis has countless advantages including high efficiency, increased stability, high degree of selectivity (regio-, chemo-, and enantio-selectivity), and safe reaction conditions [6,7]. α,!-Diols are used as monomers for the production of polyesters and polyurethanes [1,2]. At present, industrial-scale preparation has only been confined to short-chain diol biosynthesis, while biosynthesis of medium- to long-chain α,!-diols (C8–C16) has not been studied extensively [8,9]. Microbial synthesis of α,!-diol (>C12) has been originally reported in some yeasts species belonging to genus Candida [10–12]. Further microbial synthesis α,!-diols (C5–C12) from alkanes employing cytochrome P450 (CYP5153A) from Acinetobacter sp. OC4 in Escherichia coli with the highest yielded for 1,8-octanediol (722 mg/L) has been reported [10,13]. Similar ability of producing α,!-diols from alkanes through in vitro biocatalytic reactions using CYP153A16 from Mycobacterium merinum and CYP153A34 from Polaromonas sp. strain JS666 has been reported. CYP153A16 produced C8–C12 α,!-diols while CYP153A34 produced C8–C9 α,!-diols. However, the overall yield of α,!-diols from 1 mM substrate is only 3–12% [14]. Only one study has reported the use of renewable medium-chain fatty alcohols for the production of α,!3-diols Catalysts 2018, 8, 4; doi:10.3390/catal8010004 www.mdpi.com/journal/catalysts CatalystsCatalysts 2018, 8, 4 22 ofof 1111 for the production of α,ω3‐diols in E. coli by employing engineered P450BM3 [9]. Microbial synthesis inof E.medium coli by‐ employingto long‐chain engineered α,ω‐diols P450BM3 suffers major [9]. Microbial challenges synthesis such as of low medium- product to yields, long-chain low αproductivities,,!-diols suffers and major narrow challenges substrate such as lowrange product of yields,enzymes low productivities,used [9,13,15]. and Although narrow substrate some rangepreparative of enzymes‐scale usedreactions [9,13 ,15have]. Although been reported some preparative-scale [13], novel approaches reactions are have highly been reporteddesirable [13 to], novelovercome approaches bottlenecks are highlyin microbial desirable synthesis to overcome of α,ω‐diols. bottlenecks In this light, in microbial here we synthesis report highly of α, !efficient-diols. Inand this sequential light, here biocatalytic we report conversion highly efficient of free and fatty sequential acids (FFAs) biocatalytic to medium conversion‐ to long of‐chain free fatty α,ω‐diols. acids (FFAs)Vegetable to medium- oil derivatives to long-chain (e.g.,α,! FFAs)-diols. are important renewable resources [1,2]. Unfortunately, limitedVegetable attention oil has derivativesbeen paid to (e.g., synthesize FFAs) diols are from important FFAs that renewable can serve as resources good starting [1,2]. Unfortunately,materials for green limited processes, attention including has been the paid production to synthesize of α, diolsω‐diols from [13,15]. FFAs We that describe can serve herein as good the startingproduction materials of medium for green‐ to processes, long‐chain including α,ω‐diols the from production FFAs using of α,! CYP153A-diols [13 ,15monooxygenase,]. We describe hereincarboxylic the production acid reductase of medium- (CAR) and to long-chain E. coli endogenousα,!-diols aldehyde from FFAs reductases using CYP153A (ALRs) monooxygenase, (Figure 1). First, carboxylichydroxylation acid reductaseof FFAs (CAR)was carried and E. colioutendogenous using CYP153A33 aldehyde reductasesfrom Marinobacter (ALRs) (Figure aquaeolei1). First,(MaqCYP153A33), hydroxylation a promising of FFAs was biocatalyst carried due out to using its exceptional CYP153A33 regioselectivity from Marinobacter (>95%) aquaeolei for the (MaqCYP153A33),ω‐position [16]. Next, a promising a catalytically biocatalyst efficient due carboxylic to its exceptional acid reductase regioselectivity (CAR) from (>95%) Mycobacterium for the !marinum-position (MmCAR) [16]. Next, [17] a catalytically recently reported efficient was carboxylic used for acid selective reductase reduction (CAR) from of carboxylicMycobacterium acid marinumfunctional(MmCAR) group of [17 ω‐] recentlyhydroxy reported FFAs wasto corresponding used for selective aldehydes. reduction ofIt carboxylicexhibited acidhigh functional catalytic groupefficiency of ! (kcat/Km)-hydroxy FFAs against to corresponding benzoic acid and aldehydes. the C6–C It16 exhibited fatty acids high [17]. catalytic However, efficiency microbial (kcat/Km) hosts againstsuch as benzoicE. coli genome acid and encoded the C6–C for16 44fatty endogenous acids [17]. aldehyde However, reductases microbial (ALRs), hosts such and as amongE. coli genomethem 13 encodedare highly for active 44 endogenous that converted aldehyde aldehydes reductases to alcohols (ALRs), ranging and among from C them2 to C 1318 are[18,19]. highly To active efficiently that convertedconvert ω‐ aldehydeshydroxy fatty to alcohols aldehydes ranging to α from,ω‐diols, C2 to E. C coli18 [ 18cells,19 ].as To a efficientlysource of endogenous convert !-hydroxy ALRs were fatty aldehydesused. to α,!-diols, E. coli cells as a source of endogenous ALRs were used. 8 16 FigureFigure 1.1. SchematicSchematic diagramdiagram showingshowing the the biosynthesis biosynthesis of of medium- medium‐ toto long-chain long‐chainα α,!,ω‐-diolsdiols (C (C8–C–C16) fromfrom free free fatty fatty acids acids (FFAs). (FFAs).!-OHFAs, ω‐OHFAs,!-hydroxy ω‐hydroxy fatty acids; fatty! -OHFAlds,acids; ω‐OHFAlds,!-hydroxy ω‐ fattyhydroxy aldehydes. fatty aldehydes. 2. Results and Discussion 2. Results and Discussion 2.1. Establishment of MmCAR Reaction System 2.1. Establishment of MmCAR Reaction System CARs need to undergo posttranslational activation via phosphopantetheinylation using phosphopantetheinylCARs need to transferaseundergo posttranslational (PPTase) [17,20–22 ].activation Therefore, via MmCAR phosphopantetheinylation was co-expressed (Figure using S1) withphosphopantetheinyl surfactin phosphopantetheinyl transferase (PPTase) transferase [17,20–22]. (Sfp), Therefore, a PPTase MmCAR from Bacillus was co subtilis.‐expressedSDS-PAGE (Figure analysisS1) with surfactin confirmed phosphopantetheinyl the production of MmCARtransferase and (Sfp), Sfp a PPTase recombinant from Bacillus proteins subtilis. in soluble SDS‐PAGE form ◦ (Figureanalysis S2). confirmed Whole-cell the production (0.3 gCDW of/mL) MmCAR reaction and Sfp was recombinant carried out proteins at 30 Cin andsoluble 200 form rpm (Figure using MmCARS2). Whole with‐cell or (0.3 without gCDW/mL) co-expressing reaction was Sfp carried cells in out potassium at 30 °C and phosphate 200 rpm buffer using (100 MmCAR mM, pHwith 7.5) or inwithout the presence co‐expressing of 1% (Sfpw/ vcells) glucose in potassium and 10 mMphosphate MgCl2 buffer. Co-expressed (100 mM, cellspH 7.5) produced in the presence 9.2 mM ofof benzyl1% (w/v alcohol.) glucose However, and 10 mM cells MgCl only expressing2. Co‐expressed MmCAR cells synthesizedproduced 9.2 0.5 mM mM of of benzyl benzyl alcohol. alcohol (FigureHowever, S3). cells In both only cases, expressing only negligible MmCAR amountsynthesized of benzylaldehyde 0.5 mM of benzyl was alcohol detected (Figure (data notS3). shown). In both Thiscases, result only clearly negligible suggested amount that of Sfpbenzylaldehyde had a pivotal rolewas indetected the activation (data not of MmCARshown). This and thatresult activities clearly suggested that Sfp had a pivotal role in the activation of MmCAR and that activities of endogenous ALRs were
Details
-
File Typepdf
-
Upload Time-
-
Content LanguagesEnglish
-
Upload UserAnonymous/Not logged-in
-
File Pages11 Page
-
File Size-