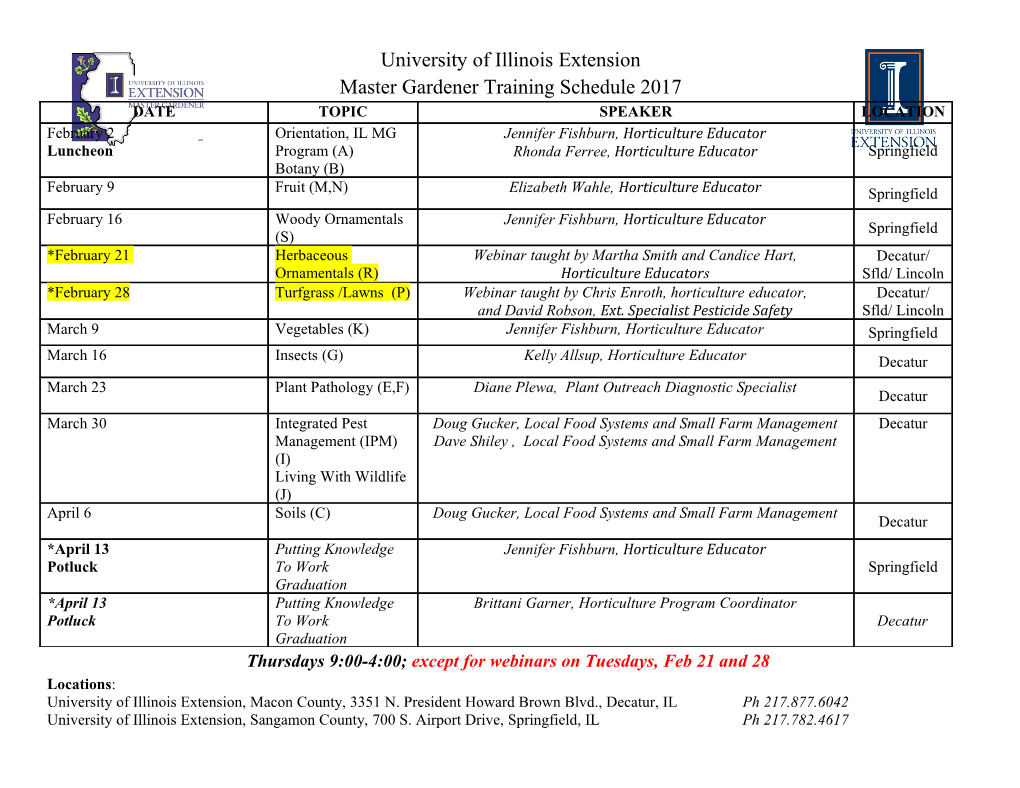
Network for Computational Nanotechnology (NCN) Atomistic Modeling of Nano Devices: From Qubits to Transistors Rajib Rahman Purdue University The Future of Electronics? Optimization: Materials & Designs New Variables: Spins ITRS New Paradigms: Quantum Logic Challenge for device modeling? New Material, Design Spintronic Devices Quantum Bits 2D Material MTJ Quantum dot Tunnel FET Spin transistor NV center Unified approach for modeling? Enter “Atoms”! Atomistic Modeling Approach Group IV & III-Vs 2D TMDs Magnetic Materials Silicon Interactions in Atomic Basis: Spin-orbit, E-field, B-field, strain Conduction electrons Donors/Acceptors Valence holes 4 Impact on Experiments Less electrons Atomistic simulations were successful to match experimental results The Future of Electronics? Optimization: Materials & Designs New Variables: Spins . Historical Perspective New Paradigms: . Exchange Interaction Quantum Logic . Spin-orbit Quantum Computing 1982: Feynman proposed a model for quantum computing. 1 Qubit: Superposition 2 Qubit: Entanglement Quantum Annealing in AQC: arXiv 1512:02206 For N qubit, all 2N states are used in parallel. 30 qubit more powerful than a supercomputer. QC: Promises massive speedup for difficult problems! History: Semiconductor Quantum Computing 1998: Loss, Divincenzo proposal - QDs 1998: Kane proposal – donors in Si Two promising proposals for semiconductor qubits! Kane’s Quantum Computer Wavefunction control (ON/OFF) Two Qubit Single Qubit Utilize Si:P for quantum computing! Wavefunction control. History: Semiconductor Quantum Computing 1998: Loss, Divincenzo proposal – QDs 1998: Kane proposal – donors in Si 2008: Single donor states observed 2005: First QD qubit in GaAs. in transport. 2012: First QD qubit in Si. Single donors probed in experiments! 2008-2012: Addressing single donors with transport Deterministic dopant location Random dopant locations Control of donor wavefunction by fields! History: Semiconductor Quantum Computing 1998: Loss, Divincenzo proposal – QDs 1998: Kane proposal – donors in Si 2005: First QD qubit in GaAs. 2008: Single donor states observed in transport. 2012: First QD qubit in Si. 2012: Deterministic single donor 2015: Two-qubit gate in Si. transistor. 2015: Realization of Kane A-gate. First electrically controlled donor qubit! 2007-2015: Implementation of Kane A-Gate Spin-orbit Stark Effect: Rahman et. Hyperfine Stark al. PRB 80, Effect 155301(2009). Expt. In Princeton Quantitative theory of donor spin control! History: Semiconductor Quantum Computing 1998: Loss, Divincenzo proposal – QDs 1998: Kane proposal – donors in Si 2005: First QD qubit in GaAs. 2008: Single donor states observed in transport. 2012: First QD qubit in Si. 2012: Deterministic single donor 2015: Two-qubit gate in Si. transistor. 2016-: Challenge: Multi-qubit gates, all 2015: Realization of Kane A-gate. electrical control 2016-: Challenge: Kane J-gate NEMO3D: Collaborations NEMO3D: Tool of choice for developed with UNSW, Delft, donor qubits. Used in UNSW. Madison, Sandia. Quantum TCAD for Qubit Design Kane J-Gate: Problem 1 B. Kane, Nature, A J A A J A 393. (1998): 133-137. Two-Qubit Gate Operation Single-Qubit Operation Large Exchange Small exchange Tunable Exchange Problem 1: Fabrication and W Control Constraints • R~15-20 nm • Gate width 20 nm • Gate cross-talk R • Possibility of J-A to be shorted Stringent fabrication constraints on 2-qubit gate Kane J-gate: Problem 2 Problem 2: J-gate tuneability is weak Need distinct ON/OFF transition Kane’s J-gate 7 times Small J-tuneability ~7 times J-gate doesn’t tune exchange much! 16 Solutions for 2-qubit gate x Kane J-gate Detuning gates E-field Reduce gate density Improve control 17 Solution: Detuning Gates (1,1) charge configuration (0,2) charge configuration Pulse from (1,1) towards (0, 2) Detuning gates ease fabrication & control Detuning based J-tuneability TCAD+NEMO+FCI 50 times 7 times A detuning gate improves J-tuneability by 1 order of magnitude in 1P-1P Boost J-tuneability even more? • STM approach 2P-1P • Detuning control • Modest field ranges (~ 4 MV/m) 5 orders of magnitude J-tuneability over Y. Wang, R. Rahman 4 MV/m in 2P-1P. Exchange Calculations: Atomistic Full Configuration Interaction Method Multi-electron Schrodinger equation in the basis of all Slater Determinants Coulomb interactions Exact solution Exchange interactions Correlations, Entanglement • General solution: Fields, interfaces i.e. device • Atomistic TB (1e – crystal+device) + FCI (e-e interaction) • Unprecedented: > 1 million atoms A. Tankasala, R. Rahman Full Configuration Interaction is an EXACT* method for many-electron problem Schemes for long distance coupling with exchange Indirect exchange: RKKY Donors coupled by dots over longer distance. Next nearest neighbor: Superexchange 3 dots: Vandersypen group 2016 Anti-ferromagnetic coupling Other types of exchange can be useful in qubits! 22 History: Semiconductor Quantum Computing 1998: Loss, Divincenzo proposal – QDs 1998: Kane proposal – donors in Si 2005: First QD qubit in GaAs. 2008: First evidence of single donor states in transport. 2012: First QD qubit in Si. 2012: Realization of deterministic 2015: Two-qubit gate in Si. single donor transistor. 2016-: Challenge: Multi-qubit gates, all 2015: Realization of Kane A-gate. electrical control, spin lifetime 2016-: Challenge: Kane J-gate Spin-orbit in QDs may enable all electrical control! Spin-orbit Coupling in Si QDs SOC in Silicon . Small in bulk Si . Non-negligible SOC near interfaces . Effects observed in Rough Surface expts. Si MOS DQD (Sandia) Understanding SOC effects observed in experiments. Valley dependent spin splitting Energy Si QD: v+ hfv+ • Vertical E-field EVS • Two low lying valley states (v+, v-) hf v- v- • Spin splitting: hfv+= gv+μB and hfv- = gv-μB B-field fv± = ESR frequencies, gv± = valley g-factor, Experiments: Spin splitting different in each valley state! 25 ESR frequency in 2 expts. Vandersypen Group (Delft): Dzurak Group (UNSW): Nature Nanotechnology 9, 666– PRB 92, 201401(R) (2015) 670 (2014) Two expts. at odds! Why? fv- Bext along [110] fv- - fv+ fv+ fv- f v+ Bext along [110] f - f <0 , f < f v- v+ v- v+ fv- - fv+ >0 , fv- > fv+ Different sign of (fv- - fv+) in two experiments 26 ESR frequency with B-field angle Vandersypen group [010] [1-10] [-110] [110] B θ fv- - fv+ >0 , fv- > fv+ [-100] [100] [-1-10] [1-10] fv- - fv+ <0 , [0-10] f < f [110] v- v+ Explain anisotropy of valley g-factors! Experimental observation: fv- - fv+ changes sign with direction of magnetic field 27 Atomistic treatment of SOC fv- - fv+ changes sign: • In different experiments (same B direction) • With B direction Valley dependence of ESR frequency/ electron g-factor: • Intrinsic spin-orbit coupling in Si Atomistic treatment of SOC k.p theory assumes various SOC terms such as Rashba and Dresselhaus. SOC in orbital basis (p orbital for Si & III-Vs) Define interface geometry & various SOCs fall out automatically. No parameters. Atomistic SOC is comprehensive & general!28 Two cases: Smooth & Rough surface Real Si devices have interface steps (interface symmetry is modified): Wave-function in ideal interface Wave-function in tilted interface Interface steps Can interface steps/roughness affect spin splitting? Calculated ESR frequency vs B-field angle tilt angle Increasing tilt angle: • more steps seen by the dot Tilt 1.1° : 1 step Tilt 1.43° : 2 steps Tilt 1.9° : 3 steps Resolution: Two expts. Have different # of steps in the dot! Interface step affects both the magnitude and sign of (fv- - fv+) 30 Atomistic SOC: comparison with experiment Vandersypen group expt. [1-10] Steps inside the dot Micro-magnet: Extrinsic SOC [110] Close agreement between theory and expt. No adjustable parameters. R. Ferdous, L. Vandersypen, R. Rahman (in prep) 31 Physics of Si QDs Si QD spin qubit atomistic effects Valley Electron g-factor physics Spin-orbit Driving properties coupling Spin lifetime Interface condition Atomistic effects influence the properties of Si QD spin qubit The Future of Electronics? Optimization: Materials & Designs New Variables: Spins New Paradigms: Quantum Logic How can we scale supply voltage? Vdd Scaling S.S < 60 log Id mv/dec S.S > 60 mv/dec VG 0 VDD Need to make the I-V curve steeper. Steepness: MOSFET vs Tunnel FET log f(E) Vg↑ log Id Hot carriers e E S D SS≥60 mV/dec Metal Oxide MOSFET n+ p n+ Vg 0 Vdd log Id log Id log f(E) No hot carriers Ef TFET S G D SS<<60 mV/dec Metal Oxide i V p+ n+ 0 dd Vg Why 2D materials for TFET? Thinner the channel shorter the tunneling distance tsi ↓ λ↓ Thick channel Thin channel S G D G Metal S D Oxide Metal Oxide Thick p+ i n+ i Thin p+ n+ High On-current in 2D TFETs 36/27 Reduced thickness is good for TFETs! Atomistic simulation of 2D TMD devices MoS2 Atomic structure Band structure Device structure 1.76 eV M Γ K DFT guided TB Models of TMDs for transport simulations Comparison among TMD TFETs How good a TMD TFET can be? Atomistic simulation results Good SS, Low On-current Small bandgap and lighter effective mass TMDs make better TFETs Solution: Dielectric Engineered TFET (DE-TFET) Illustration Comments • Combination of low- Structure k and high-k dielectrics Electric field amplification • High electric field at the tunnel junction • High On-current Atomistic Simulation Patent 2015 Result H. Ilatikhameneh, G. Klimeck, R. Rahman 39 Atom to Device: Modeling Methods Atomistic Tight binding (spin-resolved) DFT Band Structure ~<200 atoms Bandstructure, wavefunctions First principles Novel Materials Semi-empirical Method TB Material
Details
-
File Typepdf
-
Upload Time-
-
Content LanguagesEnglish
-
Upload UserAnonymous/Not logged-in
-
File Pages42 Page
-
File Size-