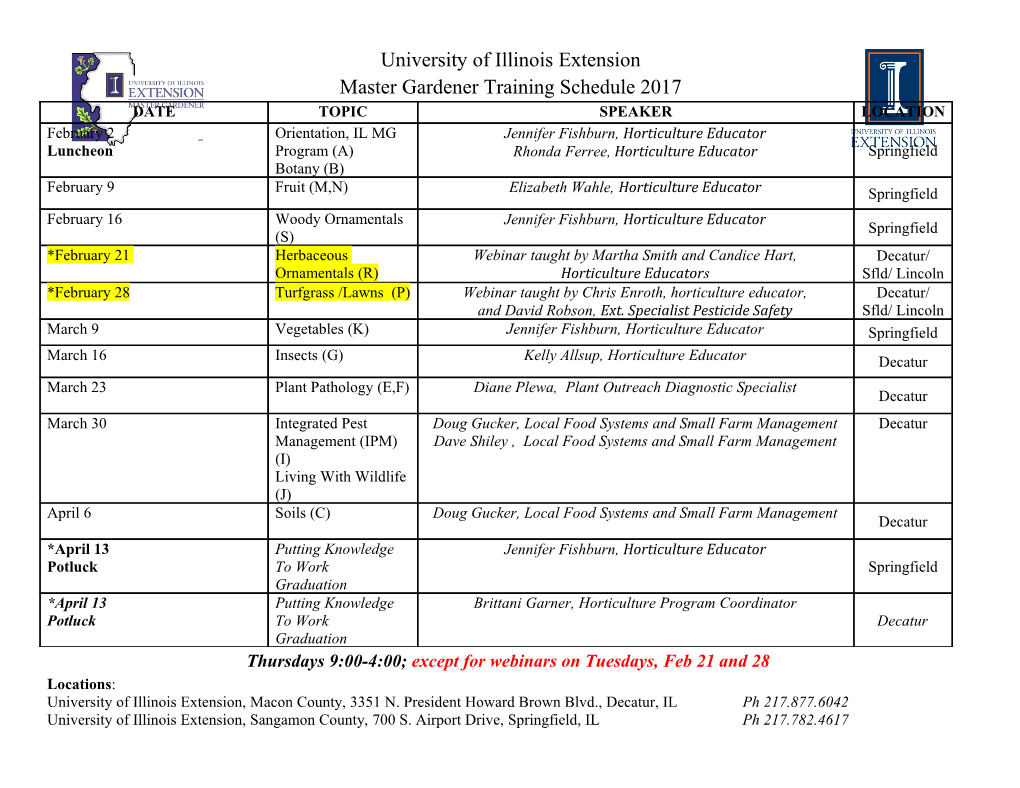
Master thesis Simple Lie algebras and singularities Nicolas Hemelsoet supervised by Prof. Donna Testerman and Prof. Paul Levy January 19, 2018 Contents 1 Introduction 1 2 Finite subgroups of SL2(C) and invariant theory 3 3 Du Val singularities 10 3.1 Algebraic surfaces . 10 3.2 The resolution graph of simple singularities . 16 4 The McKay graphs 21 4.1 Computations of the graphs . 21 4.2 A proof of the McKay theorem . 26 5 Brieskorn's theorem 29 5.1 Preliminaries . 29 5.2 Quasi-homogenous polynomials . 36 5.3 End of the proof of Brieskorn's theorem . 38 6 The Springer resolution 41 6.1 Grothendieck's theorem . 42 6.2 The Springer fiber for g of type An ............................ 45 7 Conclusion 46 8 Appendix 48 1 Introduction Let Γ be a binary polyhedral group, that is a finite subgroup of SL2(C). We can obtain two natural 2 graphs from Γ: the resolution graph of the surface C =Γ, and the McKay graph of the representation Γ ⊂ SL2(C). The McKay correspondance states that such graphs are the same, more precisely the 2 resolution graphs of C =Γ will give all the simply-laced Dynkin diagrams, and the McKay graphs will give all the affine Dynkin diagrams, where the additional vertex is the vertex corresponding to the trivial representation. 2 Γ For computing the equations of C =Γ := Spec(C[x; y] ) we need to compute the generators and Γ −1 relations for the ring C[x; y] . As an example, if Γ = Z=nZ generated by g = diag(ζ; ζ ) with −1 ζ a primitive n-th root of unity, the action on C[x; y] is g · x = ζ x and g · y = ζy (this is the contragredient representation). The invariants are u = xn; v = yn and w = xy, the unique relation 1 n is uv = w which is a singularity of type An−1. Any binary polyhedral group will give similarly a surface with an isolated singularity at the origin, called a Du Val singularity or a simple singularity. The McKay correspondance states that the resolution graph of a Du Val singularity is a Dynkin diagram and that the McKay graph of the corresponding binary group is the corresponding affine Dynkin diagram. This already gives a link between simple Lie algebras of type ADE and simple singularities, but in fact they arise naturally inside the Lie algebra itself ! More precisely, let g be a complex Lie algebra of type An;Dn;E6;E7 or E8, and G the connected, simply-connected corresponding complex Lie group. We will look at the adjoint action of G on g. This induces an action of G G on C[g] and since G is reductive, by Hilbert's theorem C[g] is finitely generated. We get a G morphism of algebraic varieties F : g ! g=G (where g=G := Spec(C[g] ) is the categorical quo- G tient) corresponding to the inclusion C[g] ⊂ C[g]. In the case of g = sln the map is simply n−1 2 3 n χ : sln ! C ;M 7! (tr(M ); tr(M );:::; tr(M )). We will see that dim g=G = r where r is the rank of G. Let x 2 g be a subregular (meaning that dim ZG(x) = r+2) nilpotent element. Let S be a transverse slice at x, that is a subvariety S of g such that the natural map TxS ⊕ Tx(G · x) ! g is ∼ surjective (we identify Txg = g). Then, if N (g) is the nilpotent cone of g, we obtain that N (g) \ S is a Du Val singularity of the same type as the Lie algebra ! This gives a connection between simple Lie algebras and simple surface singularities. Moreover, the restriction of F to S is the semiuniversal deformation of the singularity, meaning in some sense that all deformations of this singularity can be obtained by pullback of the map S ! g=G. This theorem was conjectured by Grothendieck and showed by Brieskorn for the ADE case. Slodowy extended it to Lie algebras of type BCFG. ∗ The proof of the Brieskorn's theorem uses an action of C on S and on g=G, with respect to ∗ which the quotient morphism is C -equivariant. This will give us concrete information about the morphism S ! g=G by computing the associated weights and we will be able to identify this mor- ∗ phism with the semiuniversal deformation, which also admits an action of C with the same weights. Next, we will use the Springer resolution to present a nice way of resolving the singular fibers of the map χ : g ! g=G. There is a very nice interaction between such resolutions and flag varieties. Here is how this master project is organized : in section 1 we compute the equation of the 2 surface C =Γ where Γ ⊂ SL2(C) is a finite group. In section 2 we study the geometry of such surfaces, in particular computing their minimal resolution. In section 3 we compute the McKay graphs of the binary tetrahedral groups. In section 4 we turn to Lie algebras and study the map χ : g ! g=G and its restriction to a transverse slice σ : S ! g=G, in order to prove Brieskorn's 2 theorem. In section 5, we present Springer's resolution of the nilpotent variety, which provides a nice and natural resolution of the Du Val singularities. 2 Finite subgroups of SL2(C) and invariant theory Let Γ be a finite subgroup of SL2(C). The list of such groups up to conjugacy is well known, cf [11] , chapter 1 (in fact we follow closely this chapter of the lecture notes by Dolgachev in this subsection, especially when we use relative invariants and Grundformen) or [32] for more details. By a standard averaging argument, any finite subgroup of SL2(C) is conjugate to a finite subgroup ∼ of SU2. There is a canonical map SU2 ! PSU2 = SO3, and in fact any finite group in SU2 is the pullback of a finite subgroup of SO3 (with the exception of cyclic groups of odd order) ! Later we will write explicit generators for each group, so now we just write the list : • Type An : Γ is cyclic of order n • Type Dn : Γ has order 4(n − 2), and is called the binary dihedral group of order 4(n − 2). • Type E6 : Γ has order 24, is called the binary tetrahedral group and is the pullback of A4 ⊂ SO3. • Type E7 : Γ has order 48, is called the binary octahedral group and is the pullback of S4 ⊂ SO3. • Type E8 : Γ has order 120, is called the binary icosahedral group and is the pullback of A5 ⊂ SO3. We will quickly sketch how to find this list, since the proof will also give us another useful fact concerning the orbits of the action of Γ on S2. We first claim that there is an exact sequence 1 ! {±1g ! SU2 ! SO3 ! 1 To show this, a possible way is to use that SU2 is isomorphic to the group of quaternions of norm 1, and then acts by conjugaiton on the "pure quaternions" ai + bj + ck which form a real vector ∼ 3 space V = R . In fact, it turns out that this morphism SU2 ! GL3(R) has image SO3, because 2 an element of SU2 preserve norm, and the restriction of the norm of C on V identify with the usual scalar product. Next, we claim that every finite subgroup in SU2 (except cyclic groups of odd order) is the pullback of a finite group in SO3. This can be proven by showing that finite subgroups Γ ⊂ SU2 which do not contain −1 are exactly cyclic odd subgroups. We write Γ for the image of 3 3 Γ ⊂ SU2 by the surjection SU2 ! SO3 (topologically this is the covering map S ! RP ). Now admitting the previous facts, to classify finite subgroups of SU2 we can just look at finite subgroups 3 of SO3. 2 Now let Λ a non-trivial finite subgroup of SO3. Let P be the set of points x 2 S with stabΛ(x) 6= 1. We will show the following lemma, essential for the classification : Lemma 2.1. Λ acts on P and there are only 2 orbits if Λ is cyclic, and 3 if Λ is not cyclic. Proof. Let us show that Λ acts on P : if x 2 P , that is there is h 2 Λnf1g with hx = x by definition, then for g 2 Λ(ghg−1)(gx) = ghx = gx, that is gx 2 P , so indeed Λ acts on P . 1 P g 1 If N is the number of orbits, then we have N = jΛj g2Λ jP j so N = jΛj (2(jΛj − 1) + jP j) : indeed, if g = 1 every x 2 P is fixed, else there are exactly two fixed points. Picking a representative xi in P each orbit we can write jP j = i jΛ · xij. Using that jstab(xi)j = jΛj=jΛ · xij we have 1 X 1 X 1 2 1 − = N − = 1 − jΛj jstab(x )j jstab(x )j i i i i Now, the left hand side is in [1; 2). On the other hand, the stabilizer has order at least 2 for all orbits, so we have 1 ≤ 1 − 1 ≤ 1, showing that 2 ≤ N ≤ 3. 2 |stab(xi)j If N = 2 then we obtain 2 = jP j, i.e there are two orbits in P and x1; x2 are on the same axis so Λ is cyclic.
Details
-
File Typepdf
-
Upload Time-
-
Content LanguagesEnglish
-
Upload UserAnonymous/Not logged-in
-
File Pages55 Page
-
File Size-