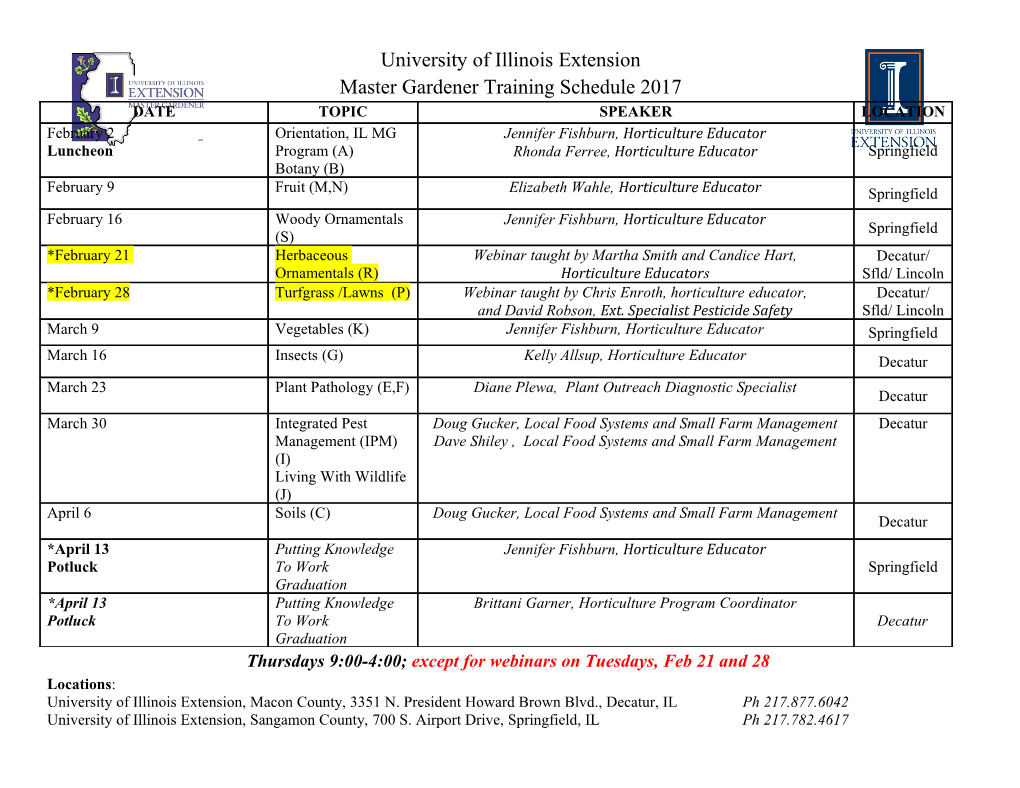
Thermal Energy Conversion Utilizing Magnetization Dynamics and Two-Carrier Effects Dissertation Presented in Partial Fulfillment of the Requirements for the Degree Doctor of Philosophy in the Graduate School of The Ohio State University By Sarah June Watzman Graduate Program in Mechanical Engineering The Ohio State University 2018 Dissertation Committee Joseph P. Heremans, Advisor Nandini Trivedi Fengyuan Yang Igor Adamovich Copyrighted by Sarah June Watzman 2018 Abstract This dissertation seeks to contribute to the field of thermoelectrics, here utilizing magnetization dynamics in two-carrier systems, employing unconventional thermoelectric materials. Thermoelectric devices offer fully solid-state conversion of waste heat into usable electric energy or fully solid-state cooling. The goal of this dissertation is to elucidate key transport phenomena in ferromagnetic transition metals and Weyl semimetals in order to positively contribute to the overarching effort of using thermoelectric materials as a clean energy source. The first subject of this dissertation is magnon drag in Fe, Co, and Ni. Magnon drag is shown to dominate the thermopower of elemental Fe from 2 to 80 K and of elemental Co from 150 to 600 K; it is also shown to contribute to the thermopower of elemental Ni from 50 to 500 K. Two theoretical models are presented for magnon-drag thermopower. One is a hydrodynamic theory based purely on non-relativistic electron-magnon scattering, and the other is based on microscopic spin-motive forces. In spite of their very different origins, the two give similar predictions for pure metals at low temperature, providing a semi-quantitative explanation for the observed thermopower of elemental Fe and Co without adjustable parameters. Magnon- drag may also contribute to the thermopower of Ni, but not dominantly as it does in Fe and Co. A spin-mixing model is presented, in which spin-up and spin-down electrons are seen as separate carriers in independent conduction channels. This model describes the magnon-drag ii contribution to the anomalous Nernst effect in Fe, again enabling a semi-quantitative match to the experimental data without fitting parameters. This work suggests that particle non-conserving processes may play an important role in other types of drag phenomena and also gives a predicative theory for improving metals as thermoelectric materials. The second subject of this dissertation is the Nernst effect in the Weyl semimetal NbP. Weyl semimetals expand research on topologically protected transport by adding bulk Berry monopoles with linearly dispersing electronic states and topologically robust, gapless surface Fermi arcs terminating on bulk node projections. Here, the Nernst effect, combining entropy with charge transport, is shown to give a unique signature for the presence of Dirac bands. The Nernst thermopower of NbP, maximum of 800 V K-1 at 9 T and 109 K, exceeds its conventional thermopower by a hundredfold and is significantly larger than the thermopower of traditional thermoelectric materials. Both the low-field and high-field Nernst coefficients also have pronounced maxima at separate temperatures. A self-consistent theory without adjustable parameters shows that this results from electrochemical potential pinning to the Weyl point energy at temperatures above the maximum temperature of the Nernst coefficients, driven by charge neutrality and Dirac band symmetry. The charge neutrality condition dictates that the Fermi level shifts with increasing temperature toward the energy that has the minimum density of states. In NbP, the agreement of the Nernst and Seebeck data with a model that assumes this minimum density of states resides at the Dirac points is taken as strong experimental evidence that the trivial (non-Dirac) bands play no role in high-temperature transport. The third subject of this dissertation, which is a study currently in progress, is the Nernst effect of YbMnBi2, a Weyl semimetal that breaks time-reversal symmetry, in the absence of an iii externally applied magnetic field. While NbP demonstrates a very large Nernst thermopower, transverse transport in that material also requires a large externally applied magnetic field, making NbP commercially unviable for use in energy generation devices. The breaking of time-reversal symmetry in YbMnBi2 gives rise to a net Berry curvature of its electronic band structure, which functions like an internal magnetic field. Here, the applied temperature gradient and measured voltage are mutually perpendicular to the predicted direction of net Berry curvature in YbMnBi2, producing a large Nernst thermopower in the absence of an externally applied magnetic field. This isothermal Nernst thermopower peaks above 1000 V K-1 at 60 K and is expected to be due directly to the material’s internal Berry curvature. The zT at 60 K is also calculated to be 2.4, exceeding the state-of-the-art zT for thermoelectric materials in the advantageous transverse geometry and eliminating the need for an externally applied magnetic field when using the transverse geometry. Nevertheless, this huge Nernst thermopower has currently only been seen on one sample; repeatability studies are in progress. Additionally, adiabatic thermomagnetic transport data presently do not align with results seen isothermally, even when parasitic temperature gradients are considered. These confirmation studies and their explanations are left in progress. iv Dedication To my mom. v Acknowledgments This work would not have been possible without the support of my advisor, Joseph P. Heremans. Thank you for being excited about new research ideas and for helping me grow into an independent researcher. I sincerely appreciate the enthusiasm with which I was met every day. One of the most important things I have learned from you is the “of course you can measure that” attitude, which forced me to be creative in developing new measurement techniques and approach projects with an open mind. Additionally, I greatly appreciate being (not so) subtly pushed in the direction of a more fundamental project, especially as an engineer. I am incredibly thankful for the instigating collaborator in my work in Weyl semimetals - Claudia Felser. I am so glad you pursued collaboration in the Weyl semimetal field with myself and my group as working with you has been a complete pleasure. I am so appreciative for your positive energy and support since the first day I met you, especially while I was a visitor in your group in Dresden, Germany. Another instrumental collaboration is that with Nandini Trivedi. I have greatly enjoyed our extensive scientific conversations, trying to understand what is actually going on and how we can explain it. Thank you for offering a theoretical point of view and explanation that pairs with my work, and thank you doing so with such passion for your work. Your support and collaboration has greatly enhanced my graduate career. vi I will forever be grateful for my labmates throughout my PhD, especially Arati, Bin, and Mike. Thank you for teaching me so much in the lab, always listening, and being my friend. Hyun and Steve – thank you for your patience and assistance as I began my PhD. Koen, Yuanhua, and Dung – thank you for always being supportive, positive, and patient. Renee – thank you for going above and beyond in everything you do. And for finding that tiny sample of NbP, thank you Koen, Bin, and Renee for crawling on the floor and sorting through dust with me. I would like to thank Tim McCormick for collaboration in the Weyl semimetal projects – thank you for all the discussions, physics explanations, and shared weary glances at our advisors. I would also like to thank the postdocs at the Max Planck Institute for Chemical Physics of Solids who helped me in every way they could to make my time there as productive as possible, especially Satya Guin, Kaustuv Manna, and Chengyuang Fu. Additionally, I would like to thank Johannes Gooth for all of his time and discussions regarding not only my data but the future of the field – thank you for bringing so much energy to this project and being supportive of my work. I would like to acknowledge my committee members, Nandini Trivedi, Fengyuan Yang, and Igor Adamovich. Thank you for your participation in this process, for supporting me, and for always being open to questions and scientific discussion. I would not have started this PhD process if it hadn’t been for encouragement from Jim Saunders and Rob Siston. Jim – thank you for identifying that graduate school was definitely for me and offering support throughout my time pursuing my PhD. Rob – thank you for guiding me vii through the twists and turns of choosing research projects and advisors, muddling through the middle of a PhD, and pursuing an academic career path. This PhD would not have been possible without the constant support and encouragement of my friends and family – thank you all for listening to more details about research than you ever wanted to hear and encouraging me to keep going. I would especially like to thank Rachel for being a constant, unending source of positivity, insight, and advice, and for listening to all of my interview talks. Joe – thank you for your infinite patience, love, and encouragement, and for always believing in me more than I believe in myself. Above all else, thank you mom and Josh. I wouldn’t have loved science, stuck with a PhD, or become the person I am today if it weren’t for you. Thank you for driving my confidence in myself and constantly reminding me that I can do anything I set my mind to throughout my entire life. You are the best. Content in Chapter 2 is published in the following: “Magnon-drag thermopower and Nernst coefficient in Fe, Co, and Ni.” S. J. Watzman, R. A. Duine, Y. Tserkovnyak, S. R. Boona, H. Jin, A. Prakash, Y. Zheng, and J. P. Heremans.
Details
-
File Typepdf
-
Upload Time-
-
Content LanguagesEnglish
-
Upload UserAnonymous/Not logged-in
-
File Pages170 Page
-
File Size-