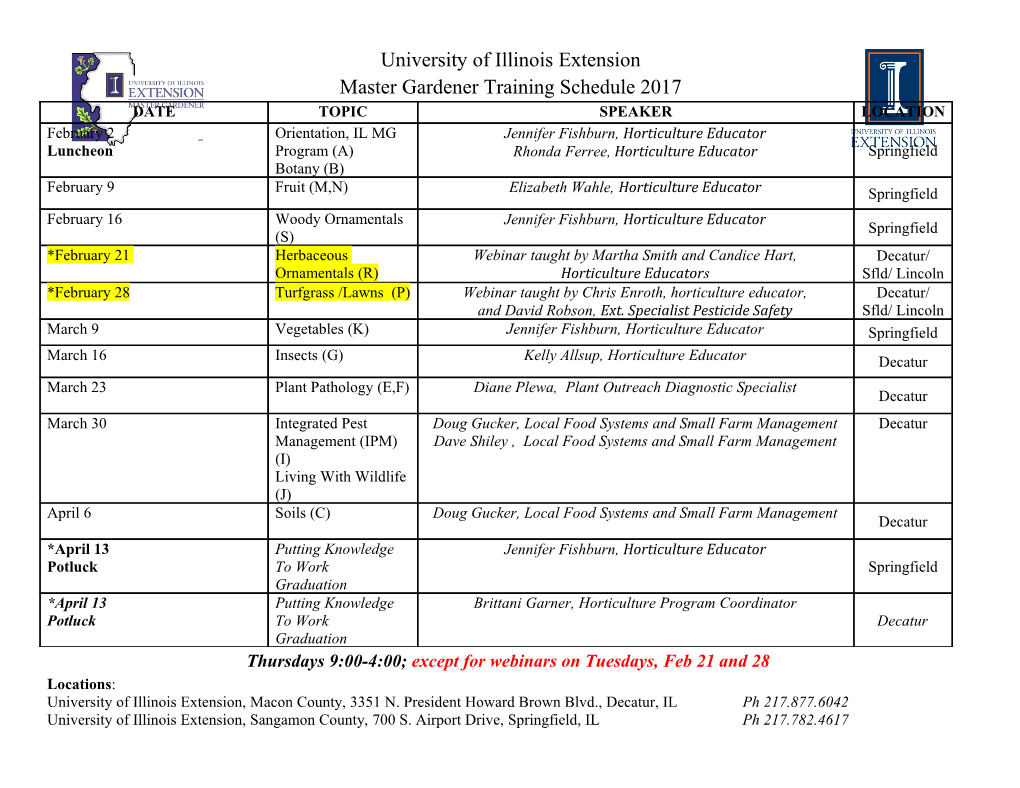
https://doi .org/10.1130/G48861.1 Manuscript received 14 October 2020 Revised manuscript received 25 January 2021 Manuscript accepted 18 March 2021 © 2021 The Authors. Gold Open Access: This paper is published under the terms of the CC-BY license. Published online 17 May 2021 Evidence for iron-rich sulfate melt during magnetite(-apatite) mineralization at El Laco, Chile Wyatt M. Bain1, Matthew Steele-MacInnis1*, Fernando Tornos2,3, John M. Hanchar3, Emily C. Creaser1 and Dorota K. Pietruszka3 1 Department of Earth and Atmospheric Sciences, University of Alberta, Edmonton, Alberta T6G 2E3, Canada 2 Instituto de Geociencias (IGEO, CSIC-UCM), Dr Severo Ochoa, 7, 28040 Madrid, Spain 3 Department of Earth Sciences, Memorial University of Newfoundland, St. John’s, Newfoundland A1B 3X5, Canada ABSTRACT GEOLOGIC CONTEXT The origins of Kiruna-type magnetite(-apatite) [Mt(-Ap)] deposits are contentious, with El Laco is a Pliocene (5.3–1.6 Ma; Naranjo existing models ranging from purely hydrothermal to orthomagmatic end members. Here, et al., 2010) stratovolcano consisting of radially we evaluate the compositions of fluids that formed the classic yet enigmatic Mt(-Ap) deposit distributed andesite flows (Naslund et al., 2002; at El Laco, northern Chile. We report evidence that ore-stage minerals crystallized from an Tornos et al., 2017) that are isotopically indis- Fe-rich (6–17 wt% Fe) sulfate melt. We suggest that a major component of the liquid was tinguishable from those of the nearby Lascar derived from assimilation of evaporite-bearing sedimentary rocks during emplacement of volcano (Matthews et al., 1994) and other vol- andesitic magma at depth. Hence, we argue that assimilation of evaporite-bearing sedimentary canoes in the Central Volcanic Zone (CVZ) of strata played a key role in the formation of El Laco and likely Mt(-Ap) deposits elsewhere. South America (Harmon et al., 1984; Richards and Villeneuve, 2001). El Laco is underlain by INTRODUCTION paragenesis of those systems (Bain et al., 2020). thick sedimentary sequences that include lime- “Kiruna-type” magnetite-(apatite) [Mt(-Ap)] This raises the possibility that carbonate-sulfate stones and sulfate evaporites of the Cretaceous– deposits (referring to the deposit at Kiruna, Swe- melts play a key role in Mt(-Ap) systems. We Tertiary Salta Group (Matthews et al., 1996; den) are major resources of iron, but their gen- test whether similar melts played a role in min- Marquillas et al., 2005; Tornos et al., 2017) and esis is vigorously debated. Some argue for a eralization at the El Laco deposit in northern phosphatic siderite ironstones of the Paleozoic hydrothermal origin, whereby veins, breccias, Chile (Fig. 1). basement (Boso and Monaldi, 1990; Mungall and replacement zones are formed by circulation The El Laco system (Fig. 1) is central to the et al., 2018). The presence of these lithologies of hot aqueous fluids of magmatic or basinal der- current debate about Mt(-Ap) genesis because it at depth likely accounts for significant crustal ivation (Hildebrand, 1986; Rhodes and Oreskes, shows (1) exceptionally well-preserved volcanic contamination of the intruding andesites (Har- 1999; Sillitoe and Burrows, 2002). Others argue textures suggesting an orthomagmatic (subaerial mon et al., 1984; Matthews et al., 1994) and the for an orthomagmatic origin, whereby orebodies volcanic) origin, and (2) widespread Na-K-Ca strong crustal Sr-Nd signature of the Mt(-Ap) are formed by emplacement and crystallization alteration suggestive of hydrothermal processes orebodies (Tornos et al., 2017). of Fe-rich silicate melt or immiscible iron-oxide (Tornos et al., 2017). Both types of features Magnetite(-apatite) mineralization at El Laco melt (Nyström and Henriquez, 1994; Naslund have been invoked as evidence for a range of occurs mostly as stratabound orebodies interbed- et al., 2002; Velasco et al., 2016; Mungall et al., contrasting orthomagmatic and hydrothermal ded with andesite flows (Naslund et al., 2002; 2018). Some models invoke combinations of models, which are difficult to reconcile. These Tornos et al., 2017). The orebodies are fed by magmatic and hydrothermal processes, includ- competing models for El Laco epitomize the magnetite-rich dikes and show prominent volca- ing exsolution of brines from Fe-rich melts wider debate about Mt(-Ap) genesis globally. nic features including flow banding, gas-escape (Tornos et al., 2017) or buoyancy-driven flota- We characterize the ore-forming fluids at El tubes, pahoehoe textures, and columnar jointing tion of magmatic magnetite by aqueous fluids Laco by detailed analyses of inclusions hosted (Henriquez and Martin, 1978; Naslund et al., (Knipping et al., 2015; Simon et al., 2018). Yet, in ore-stage diopside-magnetite-anhydrite veins 2002; Nyström et al., 2016). At Pasos Blancos, the compositions of the ore-forming fluids— from the Pasos Blancos orebody (Fig. 1; Table coarse mineralized diopside-magnetite-anhy- whether aqueous, silicate, oxide, or other— S1 in the Supplemental Material1). We focus drite (± apatite ± albite ± K-feldspar) veins remain unclear. A recent study of two Mt(- on assemblages of primary inclusions, and our (as much as 50 vol% magnetite) crosscut highly Ap) systems in the southwestern United States results show that an Fe-rich sulfate melt was altered andesite and cap subvertical lenses of showed that Fe-rich (∼4–14 wt% Fe) carbon- present during magnetite deposition. Hence, massive magnetite (Naranjo et al., 2010). The ate-sulfate melts were present throughout the we argue that sulfate-rich melts drove miner- veins show distinctive zonation, with diopside- alization at El Laco and are likely common to rich margins and anhydrite-rich interiors. Late *E-mail: [email protected] Mt(-Ap) systems more broadly. acid-sulfate and earlier alkali-calcic alteration 1Supplemental Material. Additional details on the geologic setting, analytical methods and results, Figures S1–S6, and Tables S1 and S2. Please visit https://doi .org/10.1130/GEOL.S.14470836 to access the supplemental material, and contact [email protected] with any questions. CITATION: Bain, W.M., et al., 2021, Evidence for iron-rich sulfate melt during magnetite(-apatite) mineralization at El Laco, Chile: Geology, v. 49, p. 1044–1048, https://doi.org/10.1130/G48861.1 1044 www.gsapubs.org | Volume 49 | Number 9 | GEOLOGY | Geological Society of America Downloaded from http://pubs.geoscienceworld.org/gsa/geology/article-pdf/49/9/1044/5399969/g48861.1.pdf by guest on 27 September 2021 the analyses are described in the Supplemental translucent crystals. Liquid-vapor homogeniza- A Material. Our analyses focused on coeval mag- tion is between 800 and 951 °C, overlapping the netite, diopside, and lesser albite and apatite range of T estimated from oxygen-isotope frac- from ore-stage veins at Pasos Blancos (Fig. 1C; tionation in the diopside-magnetite-anhydrite Table S1). All four minerals in all samples stud- veins (900–1125 °C; Tornos et al., 2016). ied contain assemblages of primary inclusions composed of polycrystalline aggregates of trans- DISCUSSION AND CONCLUSION lucent and opaque crystals at ambient tempera- The polycrystalline inclusions represent an ture (Figs. 2A–2C; Fig. S3 in the Supplemental Fe-rich sulfate-silicate melt (e.g., ∼6–17 wt% Material). Aqueous liquid-rich inclusions are Fe, ∼7–14 wt% Si, ∼9–11 wt% S, based on conspicuously absent from all assemblages. volumetric proportions of mineral phases pres- Phases and volumetric ratios are remarkably ent). These inclusions occur along growth zones consistent within a given assemblage and always and show consistent phase assemblages, phase B include anhydrite, hematite (5–15 vol%), and ratios, and melting behavior. Sulfate melts albite (Figs. 2D and 2H). Hematite likely rep- are stable at T as low as 900 °C in the system resents oxidation of primary magnetite owing Na2SO4-CaSO4 (Freyer et al., 1998), represented to outward diffusion of hydrogen (Mavrogenes by the subsolidus assemblage of anhydrite plus and Bodnar, 1994). Paragenetically early assem- glauberite (Fig. 2D). Addition of NaCl and H2O blages in the cores of diopside and magnetite significantly lowers the minimum melting T in grains along the vein margins are notably more this system (Walter et al., 2020, their figure 3), silica rich (based on modal proportions of and addition of silica greatly enhances sulfate phases), with anhydrite + hematite ± apatite melting down to <400 °C (Cui et al., 2020). All constituting ∼50 vol% of each inclusion, and of these factors are consistent with the inclusions albite + K-feldspar + quartz making up the observed here (Fig. 2E). Hence, the diopside- remaining ∼50 vol% (Figs. 2E–2H). Parage- magnetite-anhydrite veins were generated by netically later inclusions in the rims of diopside an Fe-sulfate-rich melt. This melt contained a grains are dominated by anhydrite (as much as significant component of felsic silicate material, ∼80 vol%), hematite, and alkali-sulfates includ- evinced by the presence of plagioclase, K-feld- ing glauberite [Na2Ca(SO4)2] (Fig. 2D). Both spar, and quartz in paragenetically earlier inclu- the earlier and later varieties of inclusions are sions (Figs. 2E–2H). This suggests that the melt primary and hosted in coeval magnetite and evolved toward a sulfate-dominant composition CDdiopside, and thus represent ore-stage fluids. over time (Figs. 2B–2D), likely as a result of Additional phases consistently observed in the fractional crystallization of silicate minerals and polycrystalline inclusions include pyrite (Fig. magnetite. S4B), natrite (Na2CO3; Fig. 2D), allanite (Fig. Anhydrite-bearing inclusions have been pre- S4), ilmenite (Fig. 2H), and halite, along with viously observed in pyroxene from hydrother- a vapor phase that occupies interstitial space mally altered andesite at El Laco and were inter- between grains (10–20 vol%; Figs. 2B and preted as “hydrous saline melts” that condensed 2C). Polycrystalline inclusions also occur along- from a hydrothermal brine (Sheets, 1997; Bro- side coeval vapor-rich inclusions in secondary man et al., 1999). The anhydrite-rich inclusions Figure 1. (A) Location of El Laco and Lascar assemblages that crosscut diopside grains.
Details
-
File Typepdf
-
Upload Time-
-
Content LanguagesEnglish
-
Upload UserAnonymous/Not logged-in
-
File Pages5 Page
-
File Size-