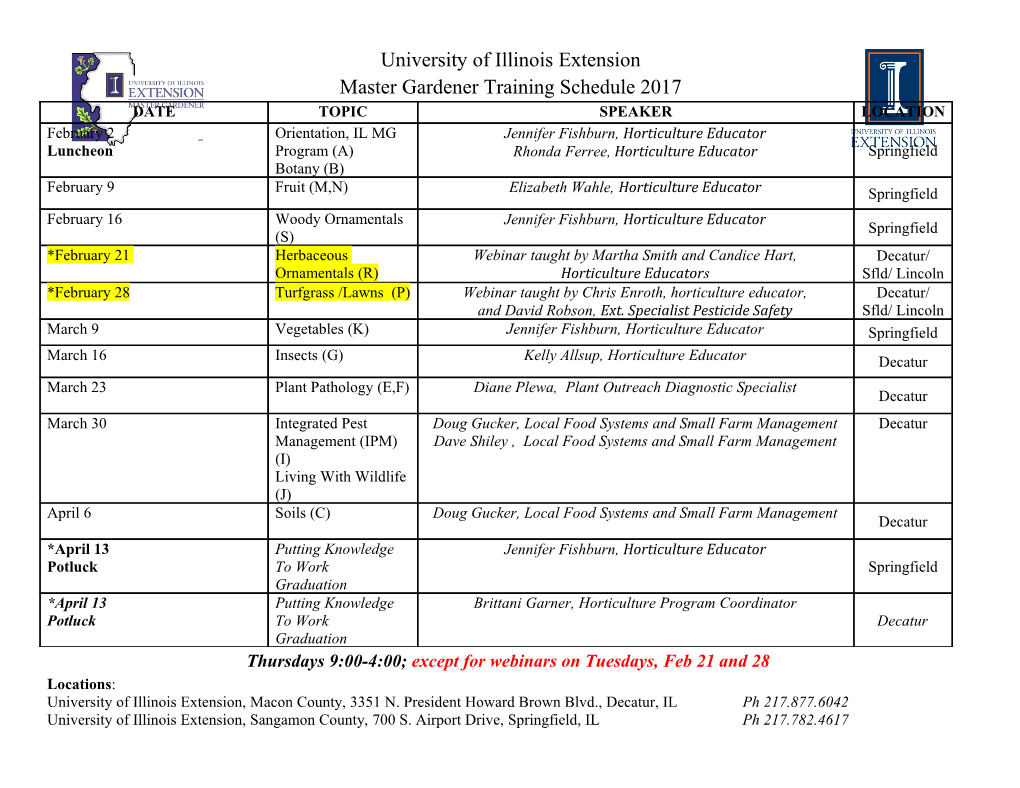
Incorporation of magnetic nanoparticles and paramagnetic molecules into human red blood cells for use as proton relaxivity enhancers for blood pool magnetic resonance imaging. Mounir Ibrahim (MSc) This thesis is presented in partial fulfilment of the requirements for the degree of the Doctor of Philosophy of The University of Western Australia School of Physics 2013 Abstract Abstract Chelated gadolinium compounds and magnetic nanoparticles have been developed for use as magnetic resonance imaging (MRI) contrast agents. However, both chelated gadolinium compounds and magnetic particles are rapidly cleared from the blood stream, gadolinium via renal excretion and magnetic nanoparticles by the reticulo-endothelial system. In order to extend the lifetime of the contrast agents in the blood stream, we have encapsulated contrast agents within red blood cells (RBCs) as a potential strategy for slowing their clearance from the blood pool. A key strategy for loading RBCs with contrast agent is to incubate them in the presence of the contrast agents under hypo-osmolar conditions. Under these conditions the cells swell and holes open up in the cell membranes allowing particles to pass into or out from the cell. The RBCs can be re-sealed by bringing the osmolarity of the medium back up to physiological values. Human RBCs were loaded with magnetic nanoparticles and gadoteric acid by two different methods. The methods comprised either hypo-osmolar incubation or a hypo-osmolar pulse in the presence of gadoteric acid or magnetic nanoparticles. The efficacy of the resulting RBCs as contrast agents for MRI was assessed by measuring the cell specific longitudinal and transverse proton relaxivities in a magnetic field of magnitude 1.4T. Human RBCs were loaded with four different magnetic iron oxide nanoparticle systems (three different polymer coated systems and one uncoated system (-Fe2O3)) using the two techniques. Polymers used comprised poly(ethylene oxide) (PEO), poly(acrylic acid) (diblock), and propylene oxide-b-ethylene oxide (PPO-b-PEO) (triblock). The aim of this study was to compare the effectiveness of magnetic resonance contrast enhancement by RBCs loaded with magnetic nanoparticles by the two different methods. Here we also tested the hypothesis that only a fraction of the RBCs in a sample open pores to enable iron oxide nanoparticles loading with each of the techniques. The optimum osmolarity for particle loading was found to be close to 200 mOsm, a condition at which the greatest fraction of particles in the incubating medium enter the cells. The maximum size of aggregate that enters the cells under 1 Abstract these conditions was found to be approximately 120 nm. Osmolarities of 150 mOsm or lower cause excessive cell destruction. At osmolarities of 200 mOsm and above, and with the osmotic pulse method, cell survival was typically about 70%. While use of uncoated magnetic nanoparticles was useful for studies of particle size limitations on cell loading, the inability to effectively wash extraneous particles from the cells makes quantitative interpretation of proton relaxivity enhancement problematical. Extraneous polymer-coated particles were easily washed from cells preparations facilitating quantitative interpretation of proton relaxometry measurements. For the polymer coated nanoparticle systems it was found that the osmotic pulse method of loading resulted in higher cell-specific transverse proton relaxivities (r2). Cell-specific transverse proton relaxivity up to 31 times higher than native red blood cells were achieved (using the triblock copolymer coated iron oxide nanoparticles introduced with the osmotic pulse technique). Human RBCs were also loaded with gadoteric acid by either hypo-osmolar incubation or a hypo-osmolar pulse in the presence of gadoteric acid. The aim of this study was to compare the effectiveness of magnetic resonance contrast enhancement by RBCs loaded with gadoteric acid by these two different methods. The resulting enhancements in proton relaxivities of cell suspensions in 1.4 T were measured and the effect of incubation osmolarity on the resulting cell specific proton relaxivity was also studied. The osmotic pulse method was found to yield the greatest cell-specific relaxivity enhancements (71-fold for longitudinal relaxivity and 39-fold for transverse relaxivity). The spatial distribution of the gadolinium within the cells was studied using energy filtered transmission electron microscopy to generate gadolinium M-edge jump ratio images. All surviving cells exposed to gadoteric acid under hypo-osmolar conditions showed enhanced (relative to control cells) and generally uniform intensity within the cells in gadolinium jump ratio images suggesting all cells are susceptible to loading and that the loading is generally spatially uniform within each cell. There was some evidence for a small amount of precipitation or aggregation of gadolinium within some cells prepared by the hypo- osmolar incubation method. 2 Table of Contents Table of contents List of Figures………………………………………. ............................................ 9 List of Abbreviations………. ................................................................................ 15 Statement of candidate contribution ...................................................................... 16 Acknowledgements ............................................................................................... 17 Chapter 1: Introduction 1.1 Magnetic Resonance Imaging development history ............................................. 18 1.2 Magnetic Resonance Imaging (Introduction) ........................................................ 19 1.2.1 MRI scanner .......................................................................................................... 21 1.3 Role of contrast agents .......................................................................................... 22 1.4 Limitations and side effects of current agents ....................................................... 23 1.5 Human blood function and red blood cells (RBCs) .............................................. 24 1.5.1 Blood function ........................................................................................................ 24 1.5.2 Red blood cells ....................................................................................................... 25 1.6 The concept of using red blood cells as a “Trojan Horse” for contrast agents ...... 27 1.6.1 Equation for manipulating osmolarity (diffusion and osmosis) ............................. 27 1.6.2 Manipulating red blood cells with osmotic pressure. ............................................ 31 1.6.3 Loading red blood cells: pore size and membrane reversibility ............................ 32 1.6.4 Previous methods of drug loading into red blood cells .......................................... 32 1.7 Aims of this study ................................................................................................... 34 Chapter 2: Theoretical Principles of MRI Contrast Agents 2.1 Basic magnetic resonance imaging theory ............................................................. 36 2.1.1 Proton magnetic relaxation .................................................................................... 39 2.1.1.1 Longitudinal (or spin-lattice) relaxation time T1 ................................................... 39 2.1.1.2 The transverse relaxation times T2 and T2* ........................................................... 40 2.1.1.3 Relationship between T2 and T2* ........................................................................... 42 2.2 Contrast agent mechanisms .................................................................................... 42 2.2.1 Ferromagnetism...................................................................................................... 44 2.2.2 Paramagnetism ....................................................................................................... 47 2.2.3 Superparamagnetism .............................................................................................. 48 3 Table of Contents 2.3 Mechanisms of longitudinal Proton Relaxation Enhancement by paramagnetic materials .......................................................................................49 2.4 Mechanisms of Transverse Proton Relaxtion Enhancement by Magnetized Particles ...................................................................................................................50 2.4.1 Static dephasing regime ...........................................................................................51 2.4.2 Motional averaging regime ......................................................................................52 2.4.3 Echo limited regime .................................................................................................52 Chapter 3: Materials and Equipments 3.1 Magnetic Nanoparticles ..........................................................................................54 3.1.1 Polymer coated Fe3O4 nanoparticles ......................................................................54 3.1.2 -Fe2O3 nanoparticles from Sigma Aldrich ............................................................54 3.1.2.1 Nanoparticle size fractionation ..............................................................................55 3.2 Gadoteric acid.........................................................................................................55 3.3 Transmission Electron
Details
-
File Typepdf
-
Upload Time-
-
Content LanguagesEnglish
-
Upload UserAnonymous/Not logged-in
-
File Pages197 Page
-
File Size-