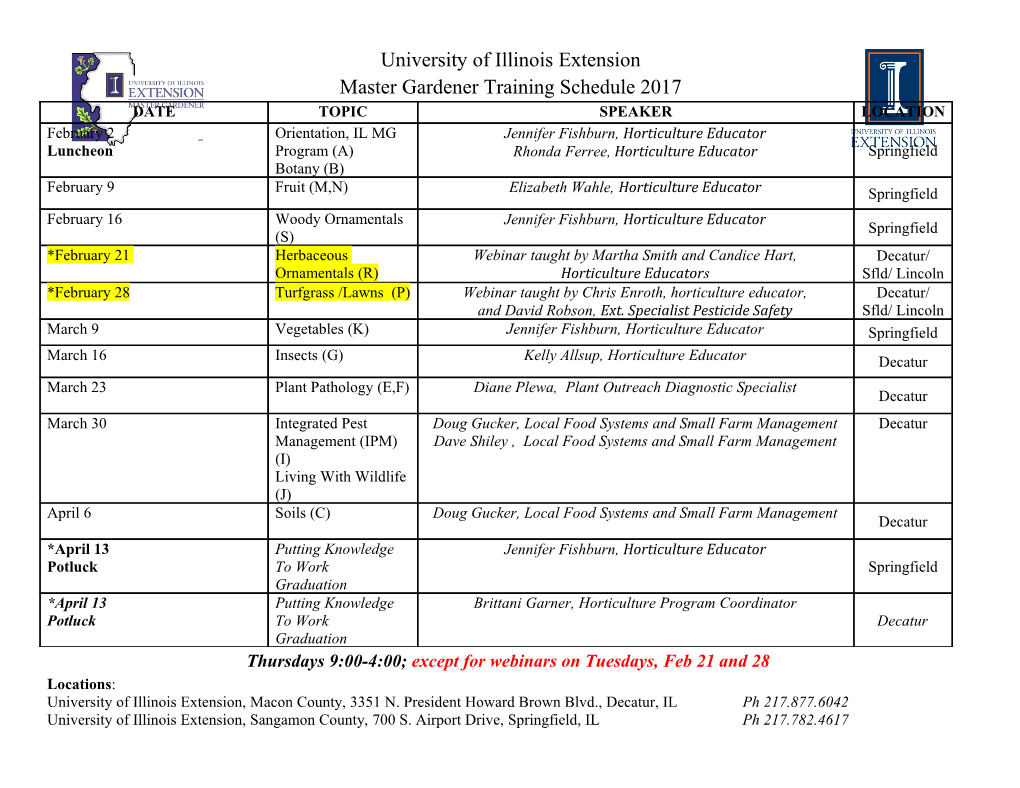
University of Groningen Secondary metabolism by industrially improved Penicillium chrysogenum strains Salo, Oleksandr IMPORTANT NOTE: You are advised to consult the publisher's version (publisher's PDF) if you wish to cite from it. Please check the document version below. Document Version Publisher's PDF, also known as Version of record Publication date: 2016 Link to publication in University of Groningen/UMCG research database Citation for published version (APA): Salo, O. (2016). Secondary metabolism by industrially improved Penicillium chrysogenum strains. University of Groningen. Copyright Other than for strictly personal use, it is not permitted to download or to forward/distribute the text or part of it without the consent of the author(s) and/or copyright holder(s), unless the work is under an open content license (like Creative Commons). The publication may also be distributed here under the terms of Article 25fa of the Dutch Copyright Act, indicated by the “Taverne” license. More information can be found on the University of Groningen website: https://www.rug.nl/library/open-access/self-archiving-pure/taverne- amendment. Take-down policy If you believe that this document breaches copyright please contact us providing details, and we will remove access to the work immediately and investigate your claim. Downloaded from the University of Groningen/UMCG research database (Pure): http://www.rug.nl/research/portal. For technical reasons the number of authors shown on this cover page is limited to 10 maximum. Download date: 28-09-2021 CCChhhaaa apppt ttteee rrr 1 1 1 1 Secondary metabolite production aapptteerr 11 by Penicillium chrysogenum hh Oleksandr Salo1, Roel A.L. Bovenberg2,3, Arnold J.M. Driessen1,4,* CC ¹Molecular Microbiology, Groningen Biomolecular Sciences and Biotechnology Institute, University of Groningen, Groningen, The Netherlands ²Synthetic Biology and Cell Engineering, Groningen Biomolecular Sciences and Biotechnology Institute, University of Groningen, Groningen, The Netherlands ³DSM Biotechnology Centre, Delft, The Netherlands ⁴Kluyver Centre for Genomics of Industrial Fermentations, Julianalaan 67, 2628BC Delft, The Netherlands Chapter 1 Submitted in modified form to Horizon Press Abstract The filamentous fungus Penicillium chrysogenum is a major industrial producer of the β-lactam antibiotic penicillin. Penicillin was discovered by Alexander Fleming in 1928, and was among the first medications to be effective against bacterial infections. Penicillins are still widely used today but are now produced by high β-lactam yielding strains that emerged from extensive classical strain improvement programs lasting for several decades. In 2008, the genome of P. chrysogenum was sequenced revealing a largely unexploited reservoir of nonribosomal peptide synthetase and polyketide synthase genes that specify potential bioactive compounds. In recent years, several pathways have been resolved that are responsible for the production of a wide variety of secondary metabolites (but these are), mostly nonribosomal peptides. Therefore, a challenge lies ahead to also elucidate the function of novel polyketide synthases and their associated products 4 Secondary metabolite production by Penicillium chrysogenum Introduction The filamentous fungus Penicillium chrysogenum belongs to the genus Penicillium and features the typical brush-shaped morphology of its conidiophores. Because of the importance of this penicillin producing fungus, it was intensive- ly studied by taxonomists during the last seven decades. Initially classified by Alexander Fleming as P. rubrum [1], this fungus has later been re-identified by Thom as P. notatum [2]. Further improvement of the taxonomical schemes resulted in association of Fleming’s isolate with the names P. chrysogenum [3], P. griseoro- seum or P. rubens [4]. The phenotypic similarities between P. chrysogenum and P. notatum were considered by Raper and Thom in 1949, who included both species into the newly established section Chrysogena (subsection Radiata). In 1977, Sam- son [3] assigned P. notatum, P. meleagrinum, P. cyaneofulvum and six addition- al species as synonymous to P. chrysogenum. Eventually, both Fleming’s isolate and the industrial penicillin producer Wisconsin were given the name Penicillium chrysogenum as nomen conservandum [5, 6]. According to the latest phylogenetic findings based on multi-gene analysis, morphological and extrolite similarities the section Chrysogena was expanded with up to eighteen species. Eight of those are producing penicillins [5]. P. chrysogenum is found in diverse environments like soil, spoiled fruits, air and even at the extreme conditions of the Antarctic ice core [7, 8]. Recently, the interest towards marine derived microorganisms as a potential source of the novel bioactive compounds, lead to the identification of P. chrysogenum species living in symbiosis with halophilic spongy Ircinia fasciculata [9] and red algal genera Lau- rencia [10]. Commonly known as non-pathogenic, P. chrysogenum has also been related to invasive human infections in patients with an immune system disorder [11]. Moreover, P. chrysogenum induces a dose-dependent allergic asthma-like re- sponse in mice [12]. The latter observation is of particular importance for human health, considering the identification of this fungus in common domestic environ- ments like old buildings, on walls and in industrial dust. 5 Chapter 1 Submitted in modified form to Horizon Press Metabolites produced by Penicillium chrysogenum During the last few decades, many bioactive compounds have been detected in the culture broth of P. chrysogenum and for several of these compounds the genes involved in their biosynthesis have been identified. Secondary metabolites are pro- duced through two main mechanisms, namely by nonribosomal peptide syntheth- ases (NRPS) and polyketide synthases (PKS). In addition, there are also hybrid NRPS-PKS enzymes. Ribosome unrelated polypeptide biosynthesis is performed by NRPS enzymes according to a thio-template mechanism. This results in poly- peptide chain assembly that is preprogrammed according to the modular sequence of the substrate specific domains within these multifunctional enzymes. The broad substrate specificity of the NRPS domains allows the incorporation of proteinogenic and non-proteinogenic amino acids as well as pseudo, hydroxy and N-methylated analogs. This provides a wide structural diversity and correspondingly broad bio- activity spectra for NRPS derived metabolites [13]. In contrast, for the biosynthesis of polyketides, CoA activated acetyl, malonyl, methylmalonyl units are required as the building blocks during extension. The produced molecules vary in length and the β-keto reduction level. Here we provide a historical overview of the secondary metabolites associated with P. chrysogenum (Fig. 1). Significant progress has been made to characterize NRPS-derived secondary metabolites but linking specific metabolites to any of the PKS-encoding genes present in the genome still lags behind. The genome of P. chrysogenum encodes 10 NPRS, 20 PKS and two hybrid NRPS-PKS genes [14]. Several of these genes have now been associated with specific products. Interest- ingly, the intensive classical strain improvement program of P. chrysogenum that was implemented from the early forties fourteens’ of last century and onwards in order to increase the yield of β-lactams eliminated pigment and other secondary metabolite formation [15]. Therefore, many of the metabolites described below are only produced in significant quantities by wild-type likeP. chrysogenum strains. The mechanisms of gene inactivation underlying this phenomenon, however, remained unresolved until now. 6 Secondary metabolite production by Penicillium chrysogenum Penicillins Penicillins were the first discovered β-lactam antibiotics that have remained ther- apeutically and commercially important for the last six decades. This class of com- pounds comprises molecules carrying a β-lactam ring nucleus within their chemical structure. β-lactam antibiotics show antimicrobial activity against Gram-positive bacteria by inhibiting cell wall biosynthesis. The β-lactam ring covalently binds to the active site of the DD-transpeptidase enzyme which is responsible for the cross- linking of the peptidoglycan bundles in the bacterial cell wall. There are three main genes involved in penicillin biosynthesis and these are clustered in the genome [16, 17]. These are the pcbAB, pcbC and penDE genes that encode the non-ri- bosomal peptide synthetase L-α-(δ-aminoadipyl)-L-α-cysteinyl-D-α-valine synthe- tase (ACVS), isopenicillin N synthase (IPNS) and isopenicillin N acetyltransferase (AT), respectively. The biosynthesis starts with the NRPS mediated condensation of α-aminoadipic acid (A), cysteine (C) and valine (V) and the formation of ACV tripeptide. β-lactam ring formation is catalyzed by Isopenicillin N (1) synthase fol- lowed by the attachment of the CoA activated side chains by Isopenicillin N acetyl- transferase. The acyltransferase enzyme also harbors hydrolytic activity, and con- verts isopenicillin N into 6-aminopenicillanic acid (6-APA). The latter is an important precursor for the production of semi-synthetic β-lactams such as amoxicillin and ampicillin. These semisynthetic production processes has expanded the activity spectrum of this class of antibiotics. Under natural conditions, a variety of side chain precursors determines the formation of the natural penicillins like, Penicillin K (2) but these
Details
-
File Typepdf
-
Upload Time-
-
Content LanguagesEnglish
-
Upload UserAnonymous/Not logged-in
-
File Pages47 Page
-
File Size-