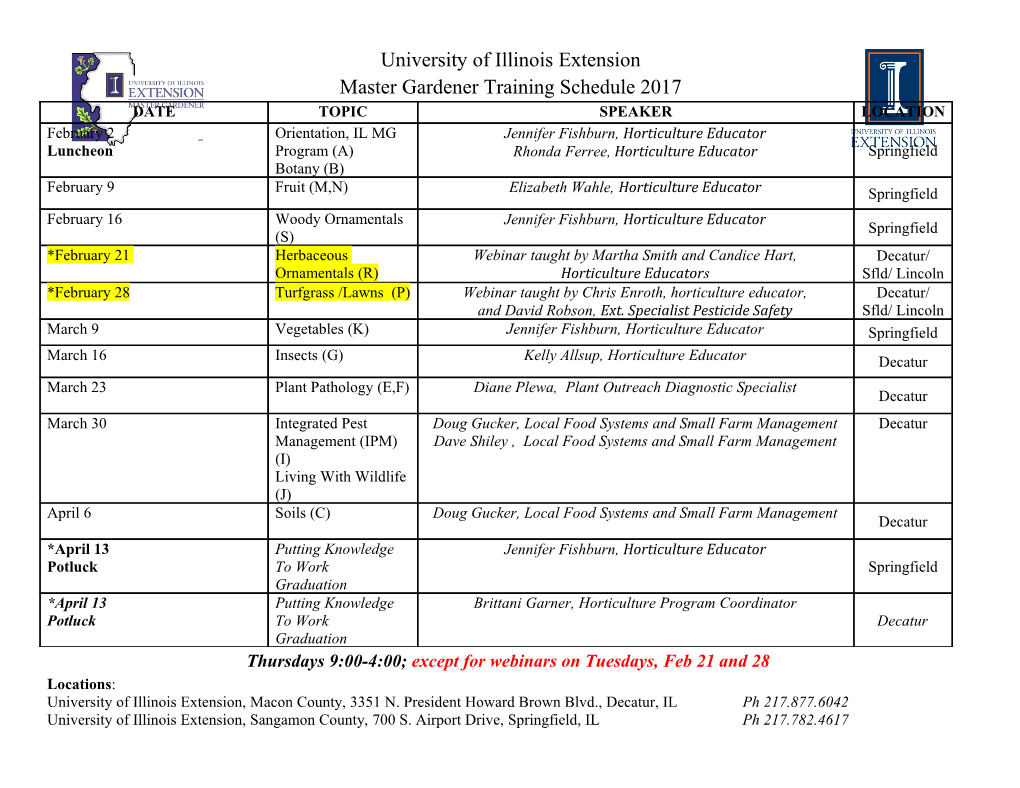
Journal of Structural and Functional Genomics 4: 67–78, 2003. 67 © 2003 Kluwer Academic Publishers. Printed in the Netherlands. Multi-domain protein families and domain pairs: comparison with known structures and a random model of domain recombination Gordana Apic1, Wolfgang Huber2 & Sarah A. Teichmann1* 1MRC Laboratory of Molecular Biology, Hills Road, Cambridge CB2 2QH, UK and 2DKFZ (German Cancer Research Center), Im Neuenheimer Feld 280, 69120 Heidelberg, Germany; * To whom correspondence should be addressed at [email protected] Received 26 November 2002; Accepted in final form 11 March 2003 Key words: structural genomics, multi-domain proteins, recombination, domain combinations Abstract There is a limited repertoire of domain families in nature that are duplicated and combined in different ways to form the set of proteins in a genome. Most proteins in both prokaryote and eukaryote genomes consist of two or more domains, and we show that the family size distribution of multi-domain protein families follows a power law like that of individual families. Most domain pairs occur in four to six different domain architectures: in isolation and in combinations with different partners. We showed previously that within the set of all pairwise domain combinations, most small and medium-sized families are observed in combination with one or two other families, while a few large families are very versatile and combine with many different partners. Though this may appear to be a stochastic pattern, in which large families have more combination partners by virtue of their size, we establish here that all the domain families with more than three members in genomes are duplicated more frequently than would be expected by chance considering their number of neighbouring domains. This duplication of domain pairs is statistically significant for between one and three quarters of all families with seven or more members. For the majority of pairwise domain combinations, there is no known three-dimensional structure of the two domains together, and we term these novel combinations. Novel domain combinations are interesting and important targets for structural elucidation, as the geometry and interaction between the domains will help understand the function and evolution of multi-domain proteins. Of particular interest are those com- binations that occur in the largest number of multi-domain proteins, and several of these frequent novel combi- nations contain DNA-binding domains. Abbreviations: SCOP: Structural Classification of Proteins database, PDB: Protein DataBank, HMM: hidden Markov model Introduction percent of eukaryote proteins are multi-domain pro- teins (Teichmann et al. 1998, Gerstein 1998a). Multi-domain proteins are the result of duplication The domains and evolutionary relationships of and combination of domains. In nature, there is a multi-domain proteins can be determined through limited number of domain families (Chothia 1992, structural annotation of genome sequences. The defi- Wolf et al. 2000), currently 1110 families of known nitions of domains and protein families for the pro- structure (Murzin et al. 1995, LoConte et al. 2002). teins of known three-dimensional structure are pro- Though the domains from these families occur on vided by the Structural Classification of Proteins their own in single-domain proteins, multi-domain (SCOP) database (Murzin et al. 1995) at the super- proteins represent the majority of proteins in a pro- family level. Based on the same sequential domain teome: two thirds of prokaryote proteins and eighty architecture, we group multi-domain proteins into 68 families, and explore the characteristics of these fam- procedure to define domain boundaries is described ilies in eighty-five genomes from the three kingdoms. in Gough et al. (2001). The domain architectures of multi-domain proteins The eighty-five completely sequenced genomes consist of sets of pairwise domain combinations from studied here have 449,823 predicted protein se- N- to C-terminus along a polypeptide chain. Previ- quences, and for more than half (241,831) of these ously, we showed that there is a limited repertoire of there is at least one structural domain assignment (Ta- domain combinations (Apic et al. 2001) and that this ble 1b) from 1041 domain families (SCOP superfam- repertoire is mainly formed by the combinatorial ver- ilies). In this work, we are interested in direct neigh- satility of a few large families. This relationship bours in a protein sequence, and so allow linkers between the size of a family and the number of dif- between domains not more than thirty residues long, ferent types of neighbouring domains in protein which is about the size of the smallest SCOP domain, sequences might suggest a stochastic basis for domain but do not consider domains that are inserted into combinations, rather than selection for particular other domains. About one half of the sequences with domain architectures. Here we show that all families structural assignments have complete assignments ac- with more than three members have fewer domain cording to this criterion, as shown in Table 1b. combinations than expected according to a random model of domain shuffling, suggesting that selection pressure is exerted to maintain the same domain pairs Families of multi-domain proteins in the evolution of domain combinations. The function of each multi-domain protein is de- We consider multi-domain proteins with the same termined by its domain composition and, in most sequential arrangement of assigned structural cases, their interactions. The protease function of chy- domains as being members of the same family. In motrypsin, for example, is carried out by the active other words, if structural domains A,B,C and D occur site buried at the interface of two domains (Sigler et in two different sequential arrangements as A-B-C-D al. 1966, Blevins et al. 1985). Therefore, elucidating and as A-B-D-C, we would form two families con- the structure of novel combinations is important in taining all the proteins with each respective domain terms of the structural and functional characterization architecture. The assumption that almost all proteins of multi-domain proteins as well as for elucidating the with the same domain architecture have descended history of protein evolution (Ponting and Russell from a common ancestor is supported by an analysis 2002). We analyze the pairwise combinations of of two-domain proteins of known structure (Bashton unknown structure and present the most popular com- and Chothia, 2001). Though many of these two- binations as targets for structural genomics projects in domain proteins had little sequence similarity, conser- the same way that individual new folds are viewed as vation of details of the sequence and structure of targets (Brenner 2001; Blundell & Mizuguchi 2000). linker regions showed that all domain pairs of the same type were related by duplications of the whole two-domain module. This is also the idea behind the Identifying domains and families Conserved Domain Architecture Retrieval Tool (Geer et al. 2002). The eighty-five genomes we study here are from the Using our definition of families of multi-domain three kingdoms of life: the fifty-five Bacteria, seven- proteins, 241,831 protein sequences with structural teen Archaea and thirteen Eukarya given in Table 1a. assignments cluster into 13,606 families of which 69 The domain assignments were taken from the are single-domain families. The size distribution of SUPERFAMILY database (Gough et al. 2001; Gough multi-domain protein families follows a power law and Chothia, 2002), which provides matches between (Figure 1) with a similar exponent to the power law the domains of the Structural Classification of Pro- of the size distribution of individual domain families teins database (SCOP, LoConte et al. 2002) and pre- (Qian et al., 2001). There is an additional pattern dicted proteins of completely sequenced genomes. within the family size distribution in that longer The method used in the SUPERFAMILY database is domain architectures tend to be less duplicated (data the iterative hidden Markov model (HMM) method not shown). In other words, longer multi-domain pro- SAM-T99 (Karplus et al. 1998), and the assignments tein families tend to have fewer members. This means described here are based on SCOP version 1.61. The that shorter domain combinations are re-used more Table 1a. 85 completely sequenced genomes used. Bacteria Archaea Eukarya Agrobacterium tumefaciens C58 Haemophilus influenzae Rickettsia prowazekii Aeropyrum pernix Anopheles gambiae Aquifex aeolicus Helicobacter pylori 26695 Salmonella typhimurium LT2 Archaeoglobus fulgidus Arabidopsis thaliana Bacillus halodurans Lactococcus lactis Shewanella oneidensis MR-1 Halobacterium sp. NRC-1 Caenorhabditis elegans rel. WS93b Bacillus subtilis Listeria innocua Sinorhizobium meliloti Methanobacterium thermoau- Ciona intestinalis 1.0 totrophicum Borrelia burgdorferi Listeria monocytogenes Staphylococcus aureus Mu50 Methanococcus jannaschii Drosophila melanogaster rel. 3 Brucella melitensis Mesorhizobium loti Streptococcus pneumoniae R6 Methanopyrus kandleri AV19 Encephalitozoon cuniculi Buchnera sp. Mycobacterium leprae Streptococcus pyogenes Methanosarcina acetivorans C2A Fugu rubripes 8.1 Campylobacter jejuni Mycobacterium tuberculosis H37Rv Streptomyces coelicolor Methanosarcina mazei Goe1 Homo sapiens 8.30 Caulobacter crescentus Mycoplasma genitalium Synechocystis sp. PCC 6803 Methanothermobacter thermau- Mus musculus
Details
-
File Typepdf
-
Upload Time-
-
Content LanguagesEnglish
-
Upload UserAnonymous/Not logged-in
-
File Pages12 Page
-
File Size-