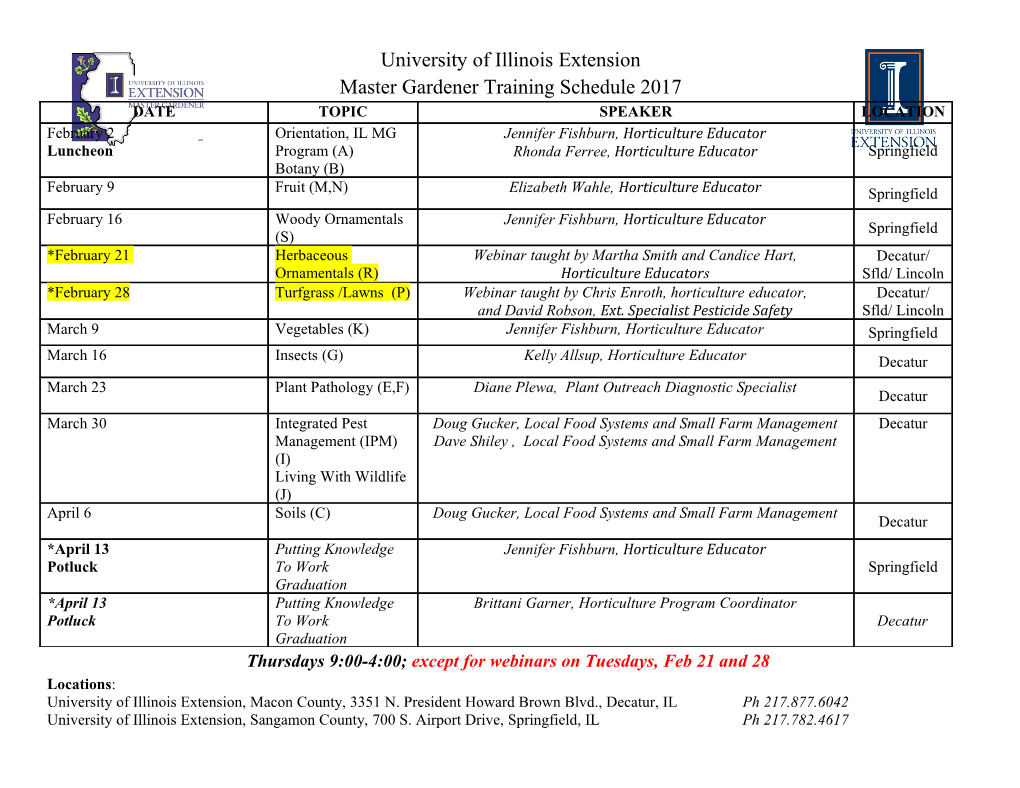
University of South Florida Scholar Commons Graduate Theses and Dissertations Graduate School November 2020 Controlling Properties of Light: Metamaterials Design and Methodology Darrick Hay University of South Florida Follow this and additional works at: https://scholarcommons.usf.edu/etd Part of the Other Education Commons Scholar Commons Citation Hay, Darrick, "Controlling Properties of Light: Metamaterials Design and Methodology" (2020). Graduate Theses and Dissertations. https://scholarcommons.usf.edu/etd/8546 This Dissertation is brought to you for free and open access by the Graduate School at Scholar Commons. It has been accepted for inclusion in Graduate Theses and Dissertations by an authorized administrator of Scholar Commons. For more information, please contact [email protected]. Controlling Properties of Light: Metamaterials Design and Methodology by Darrick Hay A dissertation submitted in partial fulfillment of the requirements for the degree of Doctor of Philosphy in Applied Physics Department of Physics College of Arts and Sciences University of South Florida Co-Major Professor: Zhimin Shi, Ph.D. Co-Major Professor: Jiangfeng Zhou, Ph.D. Libin Ye, Ph.D. Andreas Muller, Ph.D. Jing Wang, Ph.D. Date of Approval: October 23, 2020 Keywords: Metamaterials, Chirality, CPA, Polarization, Gratings Copyright c 2020, Darrick Hay Dedication To my wife Leann. You have always been there to give me motivation, console me when something didn’t work, and celebrate when it did. Without you, none of this would have been possible. To my family, for always being there when I needed you. Acknowledgments An undertaking like this is never truly the work of a single person. The list of people who have con- tributed to my work as a graduate student could more than fill a page, and I apologize if I don’t mention you by name. Know that I am forever grateful to all those that have helped, from just friendly conversation all the way to technical trainings, it has all helped shape my work. I’d first like to acknowledge the help and support given by my advisor, Dr. Zhimin Shi. Thank you for all of the interesting conversations, difficult questions, criticisms, and yes even your ruthless attention to detail. Your dedication to teaching and mentoring have shaped me into the scientist I am today. Thank you for inspiring a curiosity for optics that will last a lifetime. I’d like to thank Dr. Jiangfeng Zhou for accepting the role of co-major professor and for the discussions about metamaterials. I have a deeper understanding of device performance from our conversations. I’d like to thank the committee, Dr. Jing Wang and Dr. Andreas Muller for serving in these roles to make my dissertation of exceptional quality. Thank you to all my fellow graduate students. Corisa, Jeremy, Sookie, Ziyi, and Han, I cherish all of our conversations. You have kept me sane over the last several years. I’d like to acknowledge the support of Dr. Gerald Woods. Thank you for the opportunity to teach stu- dents who share the same love of physics that I do. Teaching these students allowed me to grow profession- ally and has enabled me to mentor future engineers and physicists that will make a meaningful contribution to society. I’d like to acknowledge all of the helpful staff at the NREC center: Robert Tufts, Richard Everly, Dr. Jay Bieber, Dr. Yusef Emirov and Sclafani Louis-Jeune, for their training, suggestions and help while I was developing the fabrication process for these metamaterials and other projects. I’d like to acknowledge the faculty within the Physics Department at USF for enabling me to accomplish this great task. The courses were stimulating and prepared me for work in industry. Last, but certainly not least, I would like to thank the Physics Department staff. Daisy, Jimmy, James, and Bobby, without you guys we would all be lost and overwhelmed. Table of Contents List of Tables . iii List of Figures . iv Abstract . vi Chapter 1 An Introduction to Metamaterials . 1 1.1 Fundamental Electromagnetic Response of Materials . .2 1.2 Negative Index Media: The Start of Modern Metamaterials . .5 1.3 Current State of Metamaterials . .8 1.4 Structure of this Thesis . .9 Chapter 2 EUV Absorber . 10 2.1 Current and Next Generation Lithography . 10 2.2 Extreme Ultraviolet Lithography and its Current Challenges . 12 2.3 EUV Materials, Multilayer Reflectors, and Absorbers . 14 2.4 Numerical Investigation of Nanoparticle Composite Absorber . 17 2.5 Application of Effective Medium Theory and Transfer Matrix Method . 22 2.6 Method for Optimizing Nanocomposite Absorbers . 23 2.7 Effects of Thinner Absorbers on the Lithography Process . 23 2.8 Conclusion . 26 Chapter 3 Chiral Metamaterials . 28 3.1 Introduction to Chiral Materials and Metamaterials . 28 3.2 90◦ Polarization Rotator in THz Regime . 31 3.2.1 Physical Mechanism Causing Chirality . 34 3.2.2 Feasibility of Fabrication . 35 3.3 Fabrication . 45 3.3.1 Preparation . 47 3.3.2 Spin Coating Polyimide . 47 3.3.3 Lithographic Patterning Process . 50 3.3.4 Electron Beam Evaporation . 51 3.3.5 Lift-Off of Photoresist . 52 3.4 Fabricated Samples . 52 3.5 Summary and Future Studies . 53 Chapter 4 Coherent Perfect Absorption in Transversely Isotropic Chiral Metamaterials . 56 4.1 Active Metamaterial Control: Coherent Perfect Absorption . 56 4.2 2-Port Scattering Matrix Theory . 58 i 4.2.1 Application of Geometric Symmetry for Transversely Isotropic Chiral Metamaterials . 62 4.2.2 Coherent Perfect Absorption in 4-fold Symmetric Chiral Materials . 66 4.3 CPA in 4-Fold Symmetric Chiral Metamaterial in the THz . 70 4.3.1 Simulation of Chiral Metamaterial in Circular Polarization Basis . 70 4.3.2 Coherent Perfect Absorption and Interferometric Polarization Control . 71 4.4 Summary and Future Work . 74 Chapter 5 Coherent Polarization Control Without Loss . 75 5.1 Background on Coherent Polarization Control Methods In Optical Regime . 75 5.2 2-Port Scattering Matrix Theory For Mirror Symmetric Structures . 76 5.3 Coherent Polarization Control in Loss-free Media . 78 5.3.1 Reconfigurable Coherent Polarization Beam Splitting . 79 5.3.2 Coherent Linear Polarization Rotation . 81 5.4 Simulating Subwavelength HICM Gratings Using RCWA . 83 5.4.1 Simulation Settings and Material Properties . 86 5.4.2 Parametric Study Results . 87 5.4.3 Broadband Coherent Polarization Control . 90 5.5 Field Profile Analysis . 98 5.6 Effective Refractive Index of HICM Gratings . 100 5.6.1 Using Bloch Theorem to Calculate Effective Material Properties . 102 5.6.2 Refractive Index Retrieval . 103 5.7 Summary . 107 References . 109 Appendix A Transfer Matrix Method . 127 A.1 Background on Transfer Matrix Theory and Electromagnetics Primer . 127 A.2 Case 1: Transverse Electric Field . 129 A.3 Case 2: Transverse Magnetic Field . 135 Appendix B Rigorous Coupled Wave Analysis . 140 B.1 Background on Rigorous Coupled Wave Analysis . 140 B.2 Case 1 Transverse Electric Polarized Fields . 142 B.2.1 Region I . 142 B.2.2 Region II . 144 B.2.3 Grating Region . 145 B.2.4 Relating the Fields using Boundary Conditions . 148 B.3 Case 2 Transverse Magnetic Polarized Fields . 150 B.3.1 Region I . 151 B.3.2 Region II . 151 B.3.3 Grating Region . 152 B.3.4 Relating the Fields using Boundary Conditions . 153 Appendix C Copyright Permissions . 155 ii List of Tables Table 1 Spin Speeds of Polyimide (PI-2525) layers. 49 Table 2 Spin Speeds of photoresist materials LOR 10B and AZ5214. 50 Table 3 The simulation ranges and sampling intervals used in RCWA simulations. 86 iii List of Figures Figure 1 Diagram showing " vs. µ and what typical materials fall within each region. .5 Figure 2 Diagram showing all reflective optics of EUV system and proposed nanocomposite absorber. 13 Figure 3 Schematic diagram describing the shadowing effect. 14 Figure 4 Reflectivity spectra comparing ML reflector, and pure Ni and TaN absorbers. 16 Figure 5 Plot of the complex index of refraction of materials at 13.5 nm . 16 Figure 6 Reflectivity spectra for all Ni-NP sizes simulated as well as the standard deviation. 19 Figure 7 Average reflectivity spectra of each Ni-NP compared with EMT and TMM method. 20 Figure 8 Farfield scatter pattern for an absorber with 2 nm, 7 nm, and no NP inclusions. 21 Figure 9 TMM calculated reflectivity as a function of absorber thicknes and fill fraction of Ni. 24 Figure 10 Calculated imaging performance of the Ni-TaN nanocomposite absorber. 26 Figure 11 Schematic diagram demonstrating the definition of chirality. 28 Figure 12 Schematic diagram of the chiral metamaterial unitcell and sandwich structure. 32 Figure 13 Reflection and transmission for the chiral metamaterial for linearly polarized inputs. 33 Figure 14 Spatial distribution of magnetic field within the CMM for fixed x and y coordinates. 36 Figure 15 Spatial distribution of magnetic field within the CMM for fixed z coordinate. 37 Figure 16 CMM reflection and transmission coefficients vs. size of wire width, gap, and cap thickness. 39 Figure 17 CMM reflection and transmission coefficients vs. wire length and spacer thickness. 40 Figure 18 Cross-polarization purity as a function of CMM geometric feature sizes. 41 Figure 19 Schematic diagram depicting layer misalignment and random line edge roughness. 43 Figure 20 Reflected and transmitted intensity as a function of layer misalignment. 44 Figure 21 CMM reflection and transmission calculated for 5 simulations of line edge roughness. 46 Figure 22 Schematic diagram representing the fabrication process for the chiral metamaterial. 48 Figure 23 Microscope image of fabricated CMM. ..
Details
-
File Typepdf
-
Upload Time-
-
Content LanguagesEnglish
-
Upload UserAnonymous/Not logged-in
-
File Pages170 Page
-
File Size-