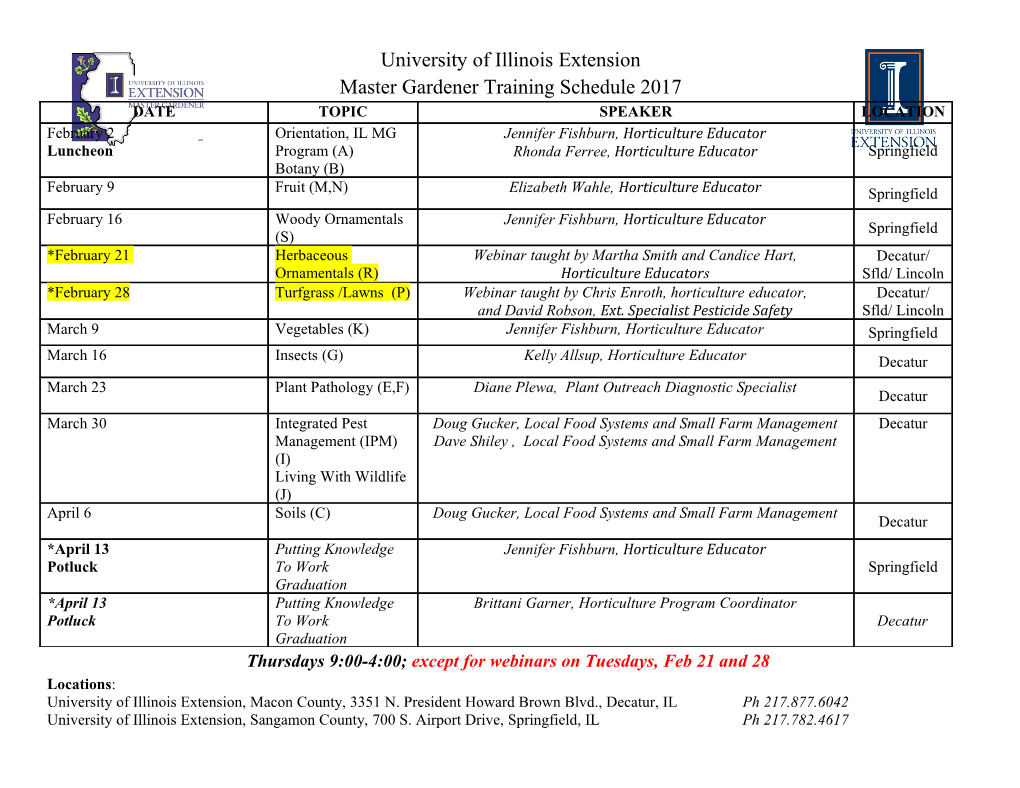
bioRxiv preprint doi: https://doi.org/10.1101/2020.09.05.284570; this version posted September 6, 2020. The copyright holder for this preprint (which was not certified by peer review) is the author/funder. All rights reserved. No reuse allowed without permission. 1 Functional metagenomics of bark microbial communities from avocado trees (Persea 2 americana Mill.) reveals potential for bacterial primary productivity 3 4 5 6 7 Eneas Aguirre-von-Wobeser 8 9 10 CONACYT – Centro de Investigación y Desarrollo en Agrobiotecnología Alimentaria, Centro de 11 Investigación y Desarrollo, A.C., Blvd. Sta. Catarina s/n, Col. Santiago Tlapacoya, 42110, San Agustín 12 Tlaxiaca, Hidalgo, Mexico 13 14 15 Correspondance: 16 Eneas Aguirre-von-Wobeser 17 52-55-25-24-86-26 18 [email protected] 1 bioRxiv preprint doi: https://doi.org/10.1101/2020.09.05.284570; this version posted September 6, 2020. The copyright holder for this preprint (which was not certified by peer review) is the author/funder. All rights reserved. No reuse allowed without permission. 19 Summary 20 21 Bark microbial communities are poorly understood, and information on the metabolic capacities of 22 their inhabitants is lacking. Bark microbial communities share part of their taxonomic composition with 23 soil, but the functional differences and similarities are unknown. By comparing bark microbial 24 communities of avocado trees (Persea americana, Mill.) with rhizospheric soil, functional processes 25 relevant to the bark environment were identified. DNA from bark and soil communities was extracted 26 from the same trees, and shotgun metagenomics sequencing was performed using nextSeq technology. 27 Genes were identified by BLAST methods, and functional annotation was performed with KEGG 28 databases as a reference. Bacterial oxygenic and anoxygenic photosynthesis genes were highly 29 abundant in bark as compared to soil. Furthermore, increased presence of nitrogenase genes suggests a 30 potential for nitrogen fixation. Genes for methanol utilization were abundant in bark, but no evidence 31 of methane utilization potential was observed. Bark microbial communities have the genetic 32 information for potential primary productivity, which might contribute to microbial growth independent 33 of plant-derived carbon substrates. 34 35 Keywords: Bark microbial communities, Metagenomics, Plant microbiome, Bacteria, Persea 36 americana (avocado), Prokaryotic photosynthesis 37 38 2 bioRxiv preprint doi: https://doi.org/10.1101/2020.09.05.284570; this version posted September 6, 2020. The copyright holder for this preprint (which was not certified by peer review) is the author/funder. All rights reserved. No reuse allowed without permission. 39 Introduction 40 41 Plants live in association with diverse communities of microorganisms, many of which have beneficial 42 or detrimental interactions with their hosts (Hassani et al., 2018), and others inhabiting plant tissues as 43 commensals. The plant microbiome is not limited to the rhizosphere, as consistent taxonomic groups 44 have been observed in aerial plant tissues as well (Lambais et al., 2014; Coleman-Derr et al. 2015; 45 Aguirre-von-Wobeser et al., 2020; Wu et al., 2020). The complexity of microbial communities on 46 aboveground plant tissues has been established by ample literature on leaf microbiology (Stone et al., 47 2018), where microorganisms are commonly found aggregated on protected grooves (Vorholt, 2012). 48 Plant surfaces are considered a challenging environment for microbial life (Stone et al., 2018), with 49 high exposure to ultraviolet radiation (UV), low availability of water, nutrient scarcity and the presence 50 of antimicrobial compounds (Vorholt, 2012). However, conclusions drawn for studies on leaves cannot 51 be directly extrapolated to the bark environment. For instance, inner stems, including the trunk of trees, 52 can be shaded by the canopy from direct UV and high photosynthtic active radiation (Muñoz-García et 53 al., 2014), which could decrease DNA damage and oxidative stress. Although water scarcity could be 54 present, its extent is not clear, as bark can store considerable amounts of water (Rosell et al., 2014) and 55 retain humidity (Mehltreter et al., 2005), which could also be aided by canopy shading. On the other 56 hand, the chemical environment in bark could be at least as challenging as the leafs (Tanase et al., 57 2019). Persea americana (Mill.) bark, for example, is known to contain compounds with bactericidal 58 activity (Akinpelu et al., 2014). 59 Adaptations to the potential stresses found on plant surfaces have been observed on the phylosphere 60 (Vorholt, 2012; Stone et al., 2018), which might be relevant on bark as well. To cope with desiccation 61 stress, bacteria can form biofilms, which can retain water (Roberson and Firestone, 1992; Gorbushina, 62 2007; Kakumanu et al., 2013). The effects of UV radiation exposure can alleviated with 63 photoprotective pigments (Jacobs et al., 2005; Vorholt, 2012) and enzymes or compounds to combat 64 oxidative stress, like catalase and superoxide dismutase or tocopherols (Sakuragi et al., 2006). 65 Nutrient scarcity is also considered a stressful condition on plant surfaces, including nitrogen limitation 66 (Vorholt, 2012; Stone et al., 2018). Nitrogenous compounds present in bark include amino acids, 67 proteins, ammonium and nitrate (O'Kennedy and Titus, 1979; Gowda et al., 1988; El-Hamalawi and 68 Menge, 1994; Wolterbeek et al., 1996; Spangenberg et al., 2002). Nitrogen fixation can also provide 69 nitrogen for some microorganisms in plant surfaces, including bark (Yatazawa et al., 1983). 3 bioRxiv preprint doi: https://doi.org/10.1101/2020.09.05.284570; this version posted September 6, 2020. The copyright holder for this preprint (which was not certified by peer review) is the author/funder. All rights reserved. No reuse allowed without permission. 70 Carbon limitation can also be severe in plant aerial surfaces. While it is recognized that carbon 71 availability is low on leaf surfaces (Vorholt, 2012; Stone et al., 2018), there could be more carbon 72 sources to support microbial growth in bark, including complex carbohydrates (Gowda et al., 1988). 73 Bacteria able to degrade and utilize complex bark carbohydrates like pectin, xylan, lignin, tannins and 74 phenolic compounds have been reported on decaying wood (Deschamps et al., 1980; Deschamps et al., 75 1983), and some have been isolated from living trees (Erakhrumen and Boyi, 2016). Transporters of 76 carbon molecules are prevalent in the phylosphere, further supporting the evidence of utilization of 77 diverse carbon substrates on plant surfaces (Lambais et al., 2017). However, the potential of plant 78 aerial surface microbial communities of acquiring carbon by photosynthesis has not been considered in 79 the literature. 80 Microbial communities of bark have similar phylum-level phylogenetic structures as the phylosphere, 81 where Proteobacteria, Actinobacteria and Bacteroidetes are dominant (Vorholt, 2012; Lambais et al., 82 2014; Leff et al., 2015; Arrigoni et al., 2018; Vitulo et al., 2019; Aguirre-von-Wobeser et al., 2020; 83 Arrigoni et al., 2020). However, it is unknown if the functional repertoires of these communities have 84 similar properties. In this work, the bark of P. americana, was analyzed, to identify metabolic networks 85 present the bacterial component of its microbial communities. Abundant prokaryotic photosynthetic 86 genes for both oxygenic and anoxygenic photosynthesis were found, as well as genes encoding 87 nitrogen fixation enzymes. These results suggest that bark microbial communities have an important 88 potential for primary productivity, which could contribute to sustain their populations in addition to 89 three-generated organic matter. 90 91 Materials and Methods 92 93 Sampling procedures 94 95 Samples collection was described previously (Aguirre von Wobeser et al., 2020). This study focuses on 96 avocado trees from an orchard located near Malinalco, Mexico (18° 59' 22.2828'' N, 99° 29' 40.4124'' 97 W). The orchard was selected to compare rhizospheric soil and bark functional potential of microbial 98 communities, as metagenomic DNA reads were available for three bark and three rhizosphere samples 99 from the same trees. A detailed description of the orchard is available (Aguirre-von-Wobeser et al., 100 2020). Briefly the orchard is located at approximately 2000 m above sea level, and is managed with 4 bioRxiv preprint doi: https://doi.org/10.1101/2020.09.05.284570; this version posted September 6, 2020. The copyright holder for this preprint (which was not certified by peer review) is the author/funder. All rights reserved. No reuse allowed without permission. 101 organic practices, with no use of agrochemicals. It was abandoned for 18 years, and recently prunned 102 and cleaned; therefore, it has a long history without agrochemical use. Weeks before sampling, a 103 preparation containing Trichoderma harzianum and Glomus sp. was added to the soil. The sampled 104 trees were located in the same of the orchard, and were healthy, productive trees. Three sample pairs of 105 bark and rhizosperic soil yielded high quality metagenomic sequences, and were included in the present 106 study. 107 108 DNA extraction, library preparation and sequencing 109 110 Total DNA was extracted with a PowerSoil kit as described in Aguirre-von-Wobeser et al. (2020). To 111 introduce bark samples to the PowerSoil kit vials, the periderm, which is the outermost layer, was 112 collected using a razor blade and cut into small pieces. Libraries were prepared with a Nextera DNA 113 Flex Library Prep Kit, using a Netxtera DNA CD Indexes kit, following manufacturer’s instructions. 114 Sequencing was performed on a nextSeq sequencer (Illumina, USA) using standard procedures. Raw 115 sequencing data can be downloaded from the NCBI Short Read Archive (BioProject PRJNA656796, 116 accession SUB7881803) and from KBase (https://kbase.us/n/69195/32/). 117 118 Bioinformatic analysis 119 120 Avocado host contamination was tested using Bowtie (Langmead et al., 2009), and was less than 2.3% 121 for all samples (Aguirre-von-Wobeser et al., 2020).
Details
-
File Typepdf
-
Upload Time-
-
Content LanguagesEnglish
-
Upload UserAnonymous/Not logged-in
-
File Pages27 Page
-
File Size-