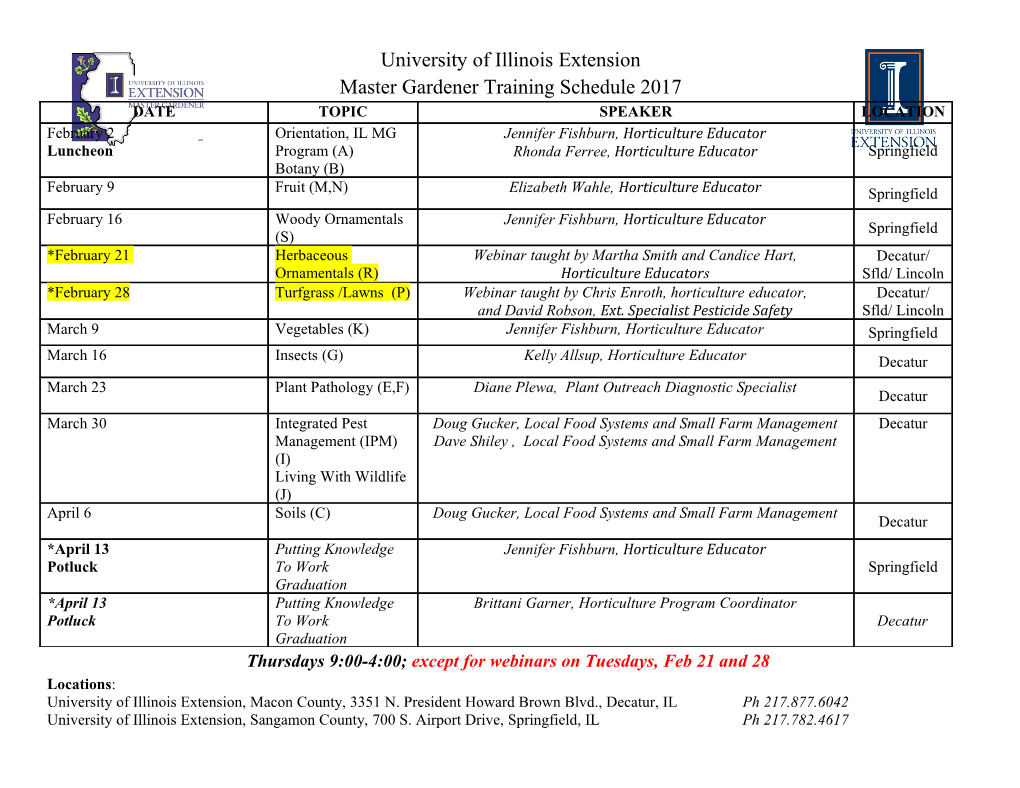
applied sciences Article A Simple and Efficient Microfluidic System for Reverse Chemical Synthesis (50-30) of a Short-Chain Oligonucleotide Without Inert Atmosphere Rahul Bhardwaj *, Phan T. Tue, Manish Biyani and Yuzuru Takamura * Department of Bioscience and Biotechnology, Japan Advanced Institute of Science and Technology (JAIST), 1-1 Asahidai, Nomi city, Ishikawa 923-1211, Japan; [email protected] (P.T.T.), [email protected] (M.B.) * Correspondence: [email protected] (R.B.); [email protected] (Y.T.); Tel.: +81-761-51-1661 Received: 7 February 2019; Accepted: 28 March 2019; Published: 31 March 2019 Abstract: Reverse DNA synthesis (50-30) plays diverse functional roles in cellular biology, biotechnology, and nanotechnology. However, current microfluidic systems for synthesizing single-stranded DNAs at a laboratory scale are limited. In this work, we develop a simple and efficient polydimethylsiloxane- (PDMS-) based microfluidic system for the reverse chemical synthesis of short-chain oligonucleotides (in the 50-30 direction) under ambient conditions. The use of a microfluidics device and anhydrous conditions effectively surpass the problem of moisture sensitivity during oligonucleotide synthesis. With optimized microfluidic synthesis conditions, the system is able to synthesize up to 21 bases-long oligonucleotides in air atmosphere. The as-synthesized oligonucleotides, without further purification, are characterized using matrix-assisted laser desorption ionization–time of flight (MALDI-TOF/TOF) mass spectroscopy (MS) supported by the denatured polyacrylamide gel electrophoresis (PAGE) analysis. This developed system is highly promising for producing the desired sequence at the nanomolar scale on-chip and on-demand in the near future. Keywords: reverse oligonucleotide synthesis (50-30), PDMS chip; on-chip and on-demand; air atmosphere 1. Introduction The past two decades have witnessed significant advancement in research efforts to incorporate synthetic short-chain ssDNAs into a variety of applications and miniaturized devices, such as oligonucleotide-Chip technology [1–5]. In the miniaturization of devices made of nucleic acids, single-stranded DNAs are the preferred choice, which are largely synthesized autonomously or non-autonomously via a staged process that uses conventional phosphoramidite chemistry [6]. The rapid growth in the use of synthetic oligonucleotides made chemical synthesis (30-50 direction) the standard protocol for preparing oligos using a commercial DNA synthesizer. This occurred because of the easy accessibility of the corresponding protected monomeric building blocks [7]. On the other hand, reverse chemical synthesis (50-30 direction) has been limited to nuclease resistance and hairpin loop applications, where the terminal linkage of natural 50-30 antisense oligonucleotides was modified via the construction of alternating 30-30 or 50-50 internucleotide linkages (terminal capping), and hence has not been matured much yet [8–11]. However, addressed micro-arrays of surface-bound ssDNAs (50-30) with a free 30 terminal group recently found multiple biotechnology applications in genetics, for example, in enzymatic extension via DNA or RNA polymerase at the 30 hydroxyl group, and transcriptomics/genomic analysis of cells using 30-poly T tail-based primers (50-30) containing short-chain oligos as a barcode address-tag (<12 bases) [12,13]. In later applications, researchers have Appl. Sci. 2019, 9, 1357; doi:10.3390/app9071357 www.mdpi.com/journal/applsci Appl. Sci. 2019, 9, 1357 2 of 13 been preparing random sequences for barcode-tags using mix and pool synthesis, which limits the application. Direct reverse synthesis of a specific sequence as an address-tag is attractive to overcome this limitation. Our work presents an application of reverse synthesis which centers upon on-chip and on-demand synthesis of a barcode as an address-tag which can be used for applications like positional information-based transcriptomics and proteomics analysis of single cells. To develop a system for the reverse synthesis of oligonucleotides, microfluidic devices were the favored choice because of their advantages like precise and reproducibly actuate fluids, low reagent consumption, and plentiful quantity production [14]. Most of the microfluidic systems have been limited to continuous flow reactors fabricated in glass or silicon because a variety of organic reagents are needed for chemical syntheses, which limits the workable complexity and reagent savings [15]. In some cases where solvents are mild, it has been possible to use integrated elastomeric material to perform batch syntheses at the nanogram scale [16,17]. Hua et al. developed a chemically resistant microreactor made of a hybrid silicon–elastomer microfluidic system, but with limited chemical characterizations of the products [18], whereas Quake et al. reported a photocurable perfluoropolyether elastomer for oligonucleotide synthesis (50-30)[19,20]. In addition, Quake et al. succeeded in developing mild reagents, which made DNA synthesis feasible on a polydimethylsiloxane- (PDMS-) based microfluidic device [21]. Despite significant advancements, the necessity of an inert atmosphere, skilled operators, and expensive controller units to perform the synthesis is still a significant challenge. Therefore, the development of an efficient system with a simple design and ample product yield under ambient conditions is necessary; this will not only reduce the cost of the process, but also facilitate on-chip and on-demand processing at a laboratory scale. In this paper, we present a simple and low-cost PDMS microfluidic system that enables the synthesis of reverse oligonucleotides (50-30) under ambient conditions suitable for on-chip and on-demand applications. The single-layered microchannel fabrication makes the microfluidic system simple and easy to use at common laboratory scales. We have also optimized the microfluidic conditions, like flow rates and flow times of the synthesis step, to achieve a high yield of the desired sequence. 2. Materials and Methods 2.1. Materials The DNA phosphoramidite monomers and controlled pore glass (CPG) (nominal particle size 110 µm, average pore size = 1000 Å) were purchased from Link Technologies Ltd. (Scotland, UK). Superdehydrated acetonitrile (water < 0.001%, 10 ppm) was purchased from Wako Pure Chemical Industries Ltd. (Osaka, Japan). A 25–30% NH4OH aqueous solution was purchased from Fluka Analytical (Munich, Germany). The (1S)-(+)-(10-camphorsulfonyl) oxaziridine (CSO) oxidizer, 5-(ethylthio)-1H-tetrazole (ETT activator, 0.25 mol/L solution in anhydrous acetonitrile), 5-(benzylthio)-1H-tetrazole (BTT activator, 0.3 mol/L solution in anhydrous acetonitrile), and anhydrous acetonitrile (MeCN) were purchased from Glen Research (Sterling, VA, USA). The SYBR gold nucleic acid gel stain was purchased from Invitrogen (Carlsbad, CA, USA). Tris-EDTA buffer (pH = 7.5) was purchased from Integrated DNA Technologies (IDT; Coralville, IA, USA). All the DNA oligonucleotides, except the ones that we synthesized from the microfluidic chips, were ordered from IDT and were purified using high-performance liquid chromatography (HPLC). Other chemicals, including tris (hydroxymethyl) aminomethane (Tris), Tris-borate-EDTA (TBE) buffer 10x, poly (vinylpyrrolidone), and TFA, were purchased from Sigma-Aldrich (Tokyo, Japan). 2.2. Fabrication of the PDMS Microfluidic Chip The chip fabrication steps were conducted in a clean room facility at JAIST (see supplementary information, Figure S1) [22]. The PDMS chip with a microchannel was developed from a defined mold structure, made of SU-8 over silicon wafers using photolithography techniques. PTFE tubes Appl. Sci. 2019, 9, 1357 3 of 13 Appl. Sci. 2018, 8, x FOR PEER REVIEW 3 of 13 (ID 1mm, New England Small Tube Co., Litchfield, NH, USA) were inserted into the inlet and outlet holesholes ofof thethe chip,chip, andand sealedsealed thethe gapgap withwith PDMS.PDMS. AnotherAnother endend ofof thethe inletinlet PTFEPTFE tubetube waswas bondedbonded withwith aa needleneedle toto introduceintroduce reagentsreagents fromfrom rubberrubber cappedcapped glass vials (see supplementary information, FigureFigure S2a).S2a). The PDMS chip consisted of a long chamberchamber (W = 2 mm, L = 10 mm, and H = 220 µm),µm), micropillarsmicropillars (W = 120 µm,µm, L = 500 µµm,m, and H = 220 µµm),m), a zigzag channel (W = 400 µµm,m, L = 60 mm, andand HH = 220 µm)µm) near the outlet, and a through-hole (2(2mm) mm) close to inlet (Figure 1 1).). TheseThese micropillarsmicropillars areare designeddesigned (the(the gapgap betweenbetween twotwo pillarspillars waswas setset toto bebe 100 µµm)m) toto traptrap thethe CPGCPG particlesparticles ofof aa 110110 µμm size.size. For CPG loading,loading, 2020 µµlL of of freshly freshly prepared CPGs slurry (60 mg, loading capacitycapacity 25–4025–40 µµmol/g)mol/g) inin 11 mLmL anhydrous anhydrous acetonitrile acetonitrile was was introduced introduced slowly slowly from from the the through-hole through-hole using using a micropipette, a micropipette, and onceand once the micro-chamber the micro-chamber was filled, was filled, CPGs wereCPGs washed were washed with fresh with anhydrous fresh anhydrous acetonitrile. acetonitrile. A column A ofcolumn porous of CPG porous particles CPG wasparticles therefore was packedtherefore inside packed the inside reaction the chamber reaction in chamber a defined in mannera defined of particlesmanner of monolayer particles monolayer (see supplementary (see supplementary information,
Details
-
File Typepdf
-
Upload Time-
-
Content LanguagesEnglish
-
Upload UserAnonymous/Not logged-in
-
File Pages13 Page
-
File Size-