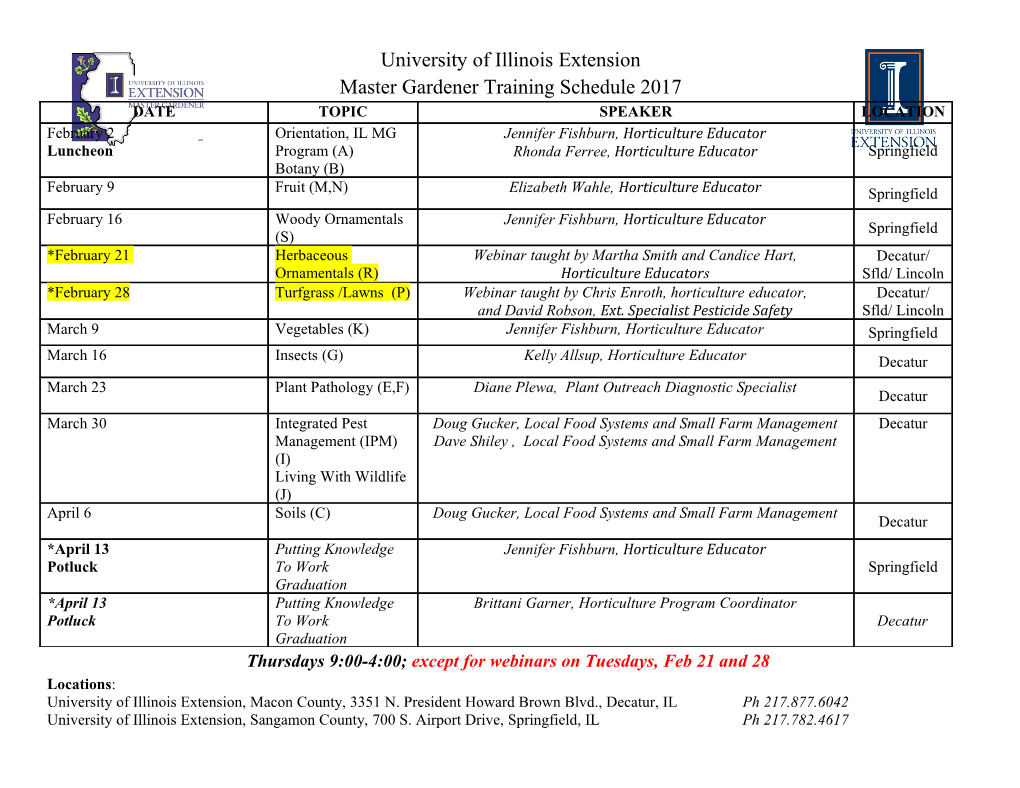
Chapter 10 Gene flow and the evolutionary ecology of passively dispersing aquatic invertebrates Beth Okamura and Joanna R. Freeland Introduction Apart from a relatively small number of ancient inland bodies of water, most fresh- water lakes originated during the last glaciation and will eventually disappear due to processes such as infilling with sediments and encroachment of marginal vegetation (Wetzel 1975). Therefore, to ensure long-term persistence, most freshwater organ- isms must at least occasionally disperse to and colonize new sites, and the wide- spread distribution of many species is indicative of such events (Darwin 1859; Pennak 1989). However, as dispersal is notoriously difficult to assess, its extent and frequency are poorly understood. This ignorance significantly compromises our understanding of both population structure and population dynamics. This chapter will review the evidence for,and assess the biological significance of, intersite dispersal and gene flow by invertebrates that inhabit lakes and ponds. We begin by broadly considering how variation in the frequency and scale of dispersal affects population and community structures. We briefly review dispersal mecha- nisms of freshwater invertebrates, and then focus on our own research programme on dispersal, gene flow and the metapopulation ecology of freshwater bryozoans. This is followed by a review of dispersal in freshwater zooplankton,an approach that allows us to compare and contrast the significance and extent of dispersal and gene flow for groups that differ in ecology and life history. Frequency and scale of dispersal Research over the last decade on the spatial ecology of populations and communities has revealed that a profound influence may be exerted by the frequency and scale of dispersal. Much of the research at the population level has focused on metapopula- tion dynamics, whereas community ecologists have focused on the relative impor- tance of regional verus local influences on patterns of species richness. Dispersal and metapopulation structure Since many organisms occur in a series of discrete and isolated sites, the metapopu- 194 195 Occupied sites Unoccupied sites Figure 10.1 Schematic representation of a classic metapopulation. Populations within local sites go extinct and are recolonized through dispersal (shown by arrows) from other currently occupied sites within the metapopulation. The pattern of site occupation varies over time with dispersal playing a crucial role in linking subpopulations within the metapopulation. lation concept (Levins 1969, 1970) has been increasingly applied by ecologists to describe the dynamics of subdivided populations. Levins’ classic metapopulation model demonstrated that, in a collection of subdivided populations, the fraction of habitat patches occupied at any one time results from a balance of the rate at which local populations go extinct and the rate of colonization of empty patches (Fig.10.1) (Hanski 1989). Since all local populations are subject to extinction, persistence ap- plies only at regional scales (Hanski & Simberloff 1997). Dispersal lies at the heart of metapopulation dynamics since it determines which habitat patches collectively comprise a metapopulation at any given time. Although metapopulation dynamics are believed to apply to many populations, conformation to the classic metapopulation model appears to be relatively rare (Harrison & Taylor 1997; Bullock et al., this volume). Further refinements of the classic model include effects such as variation in patch size or quality on extinction probabilities (e.g. island–mainland and source–sink metapopulations), the influ- ence of interspecific interactions on rates of local extinction and colonization, and spatially dependent variation in the probability of dispersal between sites (e.g. step- 196 . . ping stone and other spatially explicit models) (for reviews see Hanski & Simberloff 1997;Hanski 1999).The latter developments can account for effects of the vagility of species and landscape structure. Thus, whether a series of patches represent sepa- rate, independent populations, true metapopulations or ‘patchy populations’ (uni- tary populations within which there are discrete patches of resources; Menéndez & Thomas 2000) will be influenced by the frequency and scale of dispersal across the landscape. The frequency and scale of dispersal and accompanying gene flow will also influ- ence patterns of genetic differentiation (Slatkin 1987). Thus, patchy populations should converge towards panmixia given frequent gene flow, and there will be little genetic differentiation among local populations. At intermediate levels, gene flow will link subpopulations within a metapopulation, and moderate levels of genetic differentiation are expected due to, for example, founder effects, genetic drift and local selection. Finally, in independent populations genetic differentiation should ultimately lead to speciation. Dispersal and patterns of species richness Consideration of dispersal at the community level provides the basis for regional versus local patterns of species richness.Patterns of species composition among sites may reflect the extent to which dispersal and local interactions limit local diversity (for reviews see Cornell & Karlson 1997; Srivastava 1999). If dispersal rates greatly exceed local extinction probabilities for many species, then nearly all species in the region that are capable of invading the site should be present. Local processes will then determine species diversity and composition within patches. In this case, local species richness will approach an upper asymptote with increasing regional richness (Fig. 10.2) (Cornell & Lawton 1992). However,if dispersal is rare,then many species may be absent. Local species composition in this case will depend on a site’s history of colonization,and local interactions may be relatively unimportant (Ricklefs 1987; Cornell & Lawton 1992; Cornell & Karlson 1997). Species composition will then be undersaturated, and local species richness will increase continuously with increas- ing richness of the regional pool of species (Fig. 10.2). Such communities are con- sidered to be dispersal limited and under strong regional control. Linear, positive relationships in support of dispersal limitation have been discerned in a diversity of systems in marine, freshwater and terrestrial environments (Cornell & Karlson 1997; Srivastava 1999). How do freshwater invertebrates disperse? Intersite dispersal of freshwater invertebrates can be achieved by active or passive means (for review see Bilton et al. 2001). Active dispersal occurs through self- generated movements of organisms, mainly exemplified by aerial flight of adult in- sects. Passive dispersal is achieved by means of external agents, including floods, wind and animal vectors. Most non-insect invertebrates of lakes and ponds colonize new sites through the passive dispersal of small, dormant propagules (Williams 197 Unsaturated communities Local species richness Saturated communities Regional species richness Figure 10.2 In unsaturated communities local species richness is predicted to be a fixed proportion of regional species richness. In saturated communities,local richness may increase with regional richness but will reach an upper limit at higher regional richness. (Adapted from Srivastava 1999.) 1987). These propagules are typically highly resistant to desiccation and extremes of temperature and often show apparent adaptations to increase the likelihood of at- tachment to animal vectors. Thus, many propagules display features such as hooks, spines and sticky surfaces (Fig. 10.3); avoidance of deposition in bottom sediments through buoyancy; and accumulations of large numbers at times that coincide with peaks of waterfowl annual migrations (Bilton et al. 2001). Dispersal, life history and population genetic structure in freshwater bryozoans The life history of freshwater bryozoans Freshwater bryozoans are benthic, hermaphroditic, colonial invertebrates and are common, but overlooked, residents of freshwater habitats (Wood 1991). Their life cycle involves a high degree of clonal reproduction through colony growth by bud- ding of new zooids, colony fission or fragmentation, and the asexual production of large numbers of resistant propagules, called statoblasts. Statoblasts represent both an overwintering and dispersal stage (Fig. 10.3). Small colonies emerge from stato- blasts when favourable conditions return in the following growing season.Sexual re- production is brief in duration and results in short-lived, swimming larvae that can 198 . . Figure 10.3 Scanning electron micrograph of statoblasts of Cristatella mucedo. Scale bar = 400mm. disperse within a site before metamorphosing into a small colony. The early sea- sonal occurrence of sex in bryozoans is unusual. The majority of freshwater inverte- brate groups undergo a late season sexual phase which is viewed to be adaptive in that it results in the production of maximal genetic variation at a time that coincides with unpredictable conditions (Lynch & Spitze 1994). Thus, when conditions deteriorate,zooplankton taxa undergo a sexual phase to produce dormant,resistant embryos that will hatch out the following season. Population genetic studies of Cristatella mucedo The bryozoan Cristatella mucedo occurs as elongate,gelatinous colonies in lakes and ponds throughout the Holarctic. This widespread distribution has allowed our population genetics studies to be conducted over large spatial scales;
Details
-
File Typepdf
-
Upload Time-
-
Content LanguagesEnglish
-
Upload UserAnonymous/Not logged-in
-
File Pages23 Page
-
File Size-