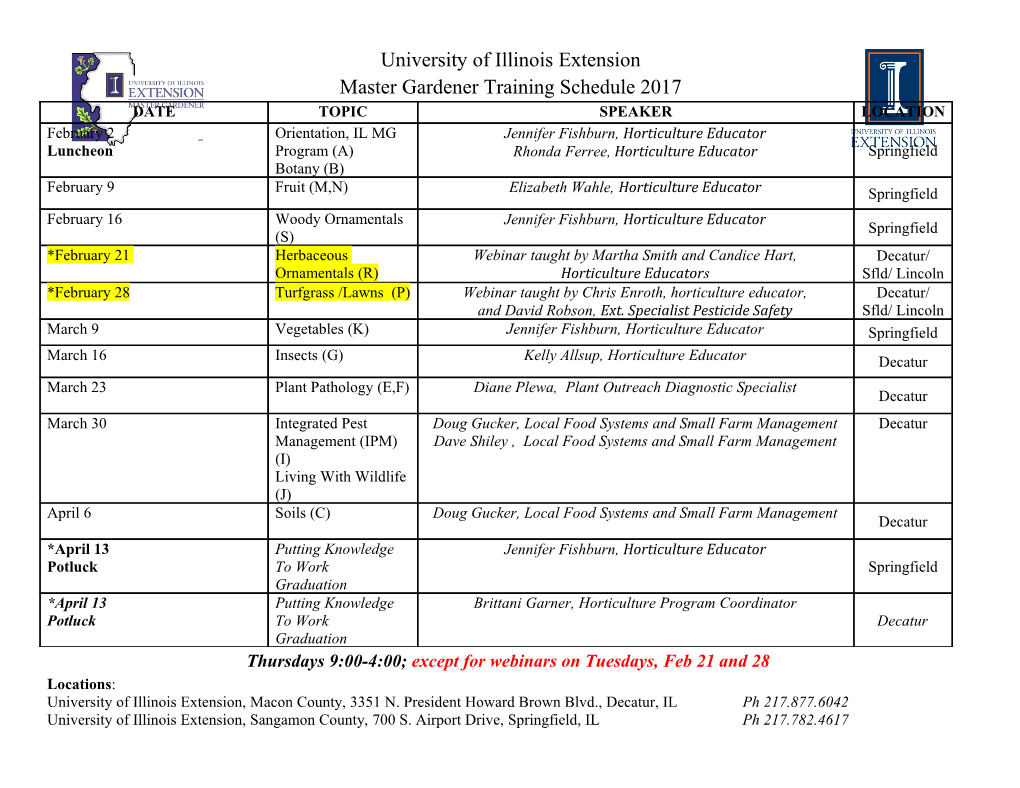
Home Search Collections Journals About Contact us My IOPscience CHARACTERIZING HABITABLE EXOMOONS This article has been downloaded from IOPscience. Please scroll down to see the full text article. 2010 ApJ 712 L125 (http://iopscience.iop.org/2041-8205/712/2/L125) The Table of Contents and more related content is available Download details: IP Address: 216.73.245.183 The article was downloaded on 02/04/2010 at 17:19 Please note that terms and conditions apply. The Astrophysical Journal Letters, 712:L125–L130, 2010 April 1 doi:10.1088/2041-8205/712/2/L125 C 2010. The American Astronomical Society. All rights reserved. Printed in the U.S.A. CHARACTERIZING HABITABLE EXOMOONS L. Kaltenegger Harvard University, 60 Garden Street, 02138 MA, Cambridge, USA; [email protected] Received 2009 December 10; accepted 2010 February 11; published 2010 March 9 ABSTRACT We discuss the possibility of screening the atmosphere of exomoons for habitability. We concentrate on Earth-like satellites of extrasolar giant planets (EGPs) that orbit in the Habitable Zone (HZ) of their host stars. The detectability of exomoons for EGPs in the HZ has recently been shown to be feasible with the Kepler Mission or equivalent photometry using transit duration observations. Transmission spectroscopy of exomoons is a unique potential tool to screen them for habitability in the near future, especially around low mass stars. Using the Earth itself as a proxy we show the potential and limits of spectroscopy to detect biomarkers on an Earth-like exomoon and discuss effects of tidal locking for such potential habitats. Key words: astrobiology – atmospheric effects – Earth – eclipses – occultations – techniques: spectroscopic 1. INTRODUCTION of a satellite from its planet is given by the Hill radius RH which depends on the distance of the planet to its host star ap,aswell Transiting planets are present-day “Rosetta Stones” for un- as the mass of the planet Mp and the star Mstar, respectively. derstanding extrasolar planets because they offer the possibility In detail, the critical semimajor axis beyond which the satellite of characterizing giant planet atmospheres (see, e.g., Tinetti would not be stable also depends on the planet’s eccentricity et al. 2007; Swain et al. 2008) and should provide access to ep as well as the satellite’s eccentricity eSat and whether it is biomarkers in the atmospheres of Earth-like bodies (Kaltenegger in a retrograde (aSat = aeR) or prograde (aSat = aeP) orbit (see, & Traub 2009; Deming et al. 2009; Ehrenreich et al. 2006), Domingos et al. 2006 for details), with additional stable regions once they are detected. Extrasolar giant planets (EGPs) might further out for periodic orbits that are not considered here (see, have “exomoon” satellites that could be detected using transit e.g., Henon 1969). The critical distance depends only weakly on time duration measurements (Sartoretti & Schneider 1999; Agol the mass ratio (Holman & Wiegert 1999), so we can safely use et al. 2005; Holman & Murray 2005; Kipping 2009), light curve the approximation of Domingos et al. (2006) which was derived distortions (Szabo et al. 2006), planet–moon eclipses (Cabrera for a mass ratio of 10−3: & Schneider 2007), microlensing (Han 2008), pulsar timing 1/3 (Lewis et al. 2008), and distortions of the Rossiter–McLaughlin RH = ap(Mp/3MStar) (1) effect of a transiting planet (Simon et al. 2009). A detailed study (Kipping et al. 2009) using transit time duration mea- surements found that exomoons around EGPs in the Habitable aeR ≈ 0.9309RH (1 − 1.0764ep − 0.9812eSat)(2) Zone (HZ) of their host star down to 0.2 Earth masses (ME)may be detectable with the Kepler Mission (Borucki et al. 1997) a ≈ 0.4895R (1 − 1.0305e − 0.2738e ). (3) or equivalent photometry, which translates into 25,000 stars eP H p Sat within Kepler’s field of view that can be screened for Earth- We calculate the critical semimajor axis for an Earth-like mass moons. This leads to the question of whether we could exomoon, for retrograde and prograde orbits around a Jupiter screen such Earth-mass exomoons remotely for habitability. mass (MJ)anda13MJ EGP in the HZ of their host star. If the viewing geometry is optimal, i.e., the exomoon is We concentrate on small stars because the transit probability p close to the projected maximum separation, the transit of an increases with decreasing stellar mass, which favors M dwarfs. exomoon is comparable to the transit of a planet the same size p ≈ Rs/ap where Rs is the radius of the star and ap is the around the star (Kaltenegger & Traub 2009), if the projected semimajor axis of the planet. The distance aHZ is defined here semimajor axis is larger than the stellar radius. In such a case, as the 1 AU-equivalent, where a planet would receive the same 0.5 transmission spectroscopy is a unique potential tool to screen stellar flux as the Earth, aHZ = 1AU(Lstar/LSun Seff) , where L exomoons for habitability in the near future. Section 2 discusses is the luminosity of the star and the normalized solar flux factor the stability and geometry of potentially habitable exomoons. Seff takes the wavelength-dependent intensity distribution of the Section 3 presents detectable spectral features of Earth-analog spectrum of different spectral classes into account, it is set to 1 exomoons. Section 4 describes our model. Section 5 presents for the Sun and 1.05 for cool stars (Kasting et al. 1993). the results and Section 6 discusses the results. 3. REMOTELY SCREENING ATMOSPHERES FOR 2. A UNIQUE GEOMETRY HABITATS Several groups have investigated the long-term dynamical Currently several exoplanets are known to have a minimum stability of hypothetical satellites and moons up to Earth masses mass below 10 ME (Mayor et al. 2009). This mass limit is orbiting EGPs (Cassidy et al. 2009; Barnes & O’Brien 2002; usually considered to separate terrestrial from giant planets, Domingos et al. 2006; Scharf 2006; Holman & Wiegert 1999) the latter having a significant fraction of their mass in a H2–He and have shown that Earth-mass moons could exist and be stable envelope. Planets and satellites without a massive H2–He for more than 5 Gyr around EGPs. The maximum stable distance envelope are potentially habitable because they may have L125 L126 KALTENEGGER Vol. 712 suitable temperatures and pressures at any solid surface to appropriate atmospheric layers. Clouds are represented by support liquid water. On Earth, some atmospheric species inserting continuum-absorbing/emitting layers at appropriate exhibiting noticeable spectral features in the planet’s spectrum altitudes. For the transit spectra, we assume that the light result directly or indirectly from biological activity: the main paths through the atmosphere can be approximated by incident ones are O2,O3,CH4, and N2O. CO2 and H2O are in addition parallel rays, bent by refraction as they pass through the important as greenhouse gases in a planet’s atmosphere and atmosphere, and either transmitted or lost from the beam by potential sources for high O2 concentration from photosynthesis single scattering or absorption. We model Earth’s spectrum (see Kaltenegger et al. 2010a, 2010b for details). using its spectroscopically most significant molecules, H2O, O3, A habitable moon would need to be able to retain its volatiles. O2,CH4,CO2, CFC-11, CFC-12, NO2, HNO3,N2, and N2O, This depends on its mass, the charged particle flux it receives, where N2 is included for its role as a Rayleigh scattering species. whether it maintains a magnetosphere, and its position with Our line-by-line radiative transfer code for the Earth has been respect to the planet’s magnetosphere, among other factors. validated by comparison to observed reflection, emission, and This leads to a lower mass limit between 0.12 ME and 0.23 ME transmission spectra (Woolf et al. 2002; Christensen & Pearl (Williams et al. 1997; Kaltenegger et al. 2000) above which 1997; Kaltenegger et al. 2007; Irion et al. 2002; Kaltenegger & moons can potentially be Earth-analog environments if their Traub 2009). For this Letter, we use an Earth model atmosphere, planet orbits within the HZ. namely the US Standard Atmosphere 1976 (COESA 1976) Our search for signs of life is based on the assumption that spring-fall model to derive the strength of the detectable feature. extraterrestrial life shares fundamental characteristics with life on Earth in that it requires liquid water as a solvent and has a 5. RESULTS carbon-based chemistry (see, e.g., Brack 1990), uses the same input and output gases and exists out of thermodynamic equi- Table 1 shows the stellar parameters, the 1 AU-equivalent librium (Lovelock 1975). “Biomarkers” is used here to mean distance aHZ, the planet’s orbital period for a 1 Jupiter mass detectable chemical species, whose presence at significant abun- P1J with an Earth-mass exomoon, and the corresponding transit dance strongly suggests a biological origin (e.g., CH4+O2,or duration T1J. The period and transit duration is reduced by less CH4+O3,N2O). “Bioindicators” (e.g., H2O, CH4) are indicative than 10% for a 13 MJ EGP orbiting the smallest host star (M9). of biological processes but can also be produced abiotically. It nT denotes the number of transits per Earth year, and σ denotes is their abundances, and their detection in the context of both the total number of hours the planet can be observed in transits other atmospheric species, and the properties of the star and per Earth year. the planet that point toward a biological origin (Kaltenegger & Table 2 shows the maximum orbital separation ae of an Selsis 2009). exomoon from its planet in stellar radii. We assume circular orbits and calculate the corresponding orbital periods Pe to 4.
Details
-
File Typepdf
-
Upload Time-
-
Content LanguagesEnglish
-
Upload UserAnonymous/Not logged-in
-
File Pages7 Page
-
File Size-