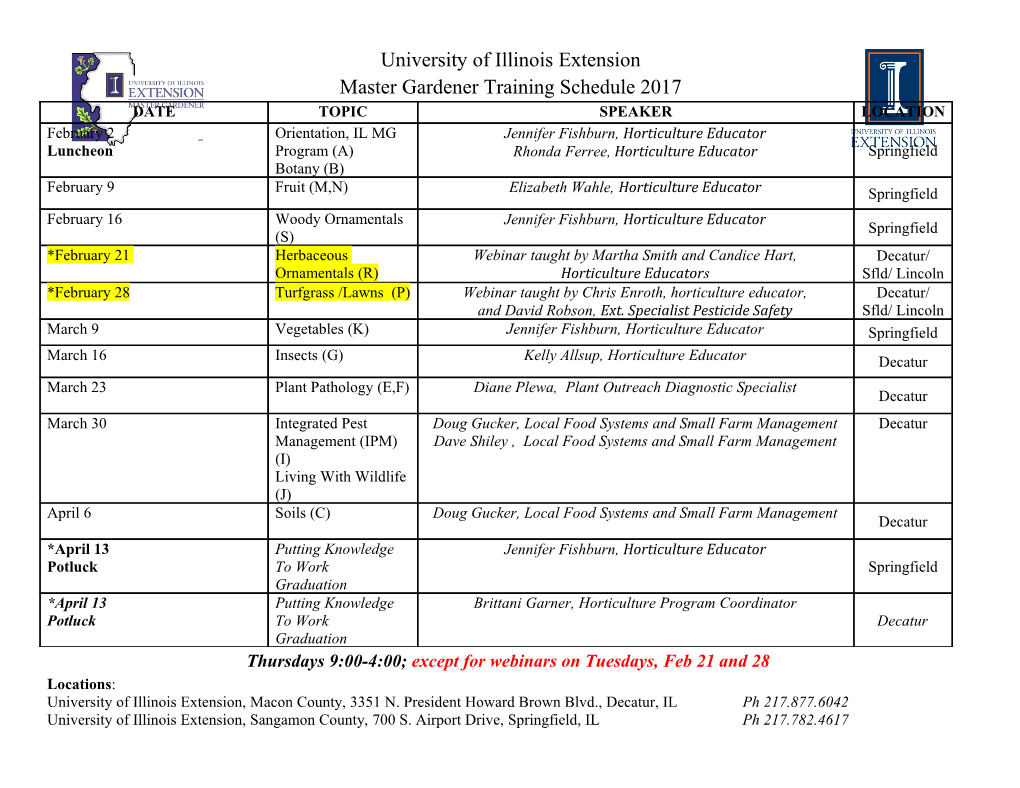
Iron sand extraction in South Taranaki Bight: effects on seawater trace metal concentrations AUT Client report: TTRL 20138 August 2013 Iron sand extraction in South Taranaki Bight: effects on seawater trace metal concentrations Kay Vopel John Robertson Peter S. Wilson Prepared for Trans–Tasman Resources Ltd. AUT Client report: TTRL 20138 August 2013 Institute for Applied Ecology New Zealand School of Applied Sciences Auckland University of Technology Private Bag 92006 Auckland 1142 Phone +64 9 921 9999 ext. 6461 www.aut.ac.nz Content Executive summary 3 Introduction 5 Material and methods 8 Approach 8 Section A 8 Section B 8 Sample collection and preparation 8 South Taranaki Bight sediment 8 Reference sediment 11 Processed sediment 11 Analyses 12 Particle size, organic matter and water content 12 Sediment extractions: AVS 12 Sediment extractions: trace metals 13 Standard elutriation 13 Standard elutriate test 14 Trace metals in sediment slurry 14 Results and discussion 15 Section A: sediment cores and sediment slurry 15 Physical properties 15 Acid volatile sulfides 17 Trace metals in sediment 17 Trace metals in sediment suspensions 19 Chromium and nickel in RC slurry 20 Section B: processed iron sand 23 Physical properties 23 Acid volatile sulfides 24 Trace metals in sediment 24 Trace metals in sediment suspensions 26 Summary and conclusions 27 References 29 Appendix I AUT Client report: TTRL 20138 2 Executive summary Trans–Tasman Resources Limited (hereafter, TTR) plans to exploit a source of vanadium-titanomagnetite in South Taranaki Bight, New Zealand, with an offshore dredge-mining operation. Because this operation will displace, modify and suspend deep anoxic sediment, it may make sediment-bound contaminants available to pelagic and benthic biota. To assess the likelihood of such effect, TTR contracted Auckland University of Technology (AUT) to conduct laboratory tests with sediment from the mining areas Christina and Diana. Specifically, TTR contracted AUT to (1) investigate if and how the sediment contents of trace metals and trace metal binding sulfides change with sediment depth, (2) investigate the release of trace metals from suspensions of this sediment, and (3) investigate the release of trace metals from suspensions of processed iron sand, that is, iron sand that has been modified by steps of magnetic separation and milling. As expected for high-energy offshore environments, the low organic matter content (<1% dry weight) of the medium sand in South Taranaki Bight explained the low sediment content of acid volatile sulfides (AVS) in both sediment-core and processed iron sand sample. We found no evidence for increases with sediment depth in sediment organic matter and AVS content. The concentrations of cadmium, chromium, copper, lead, nickel, and zinc in sediment from 0–5 m depth were below the upper ANZECC & ARMCANZ interim sediment quality guidelines concentrations (ISQG-high values, ANZECC & ARMCANZ 2000). With the exception of nickel in two of 23 sediment core samples, these concentrations were also below the lower ANZECC & ARMCANZ guideline concentrations (ISQG-low values). We infer a low probability of adverse effects of these trace metals on the functioning of the South Taranaki Bight benthic ecosystem. For all trace metals except nickel, the concentrations in standard elutriate extracts of sediment core samples were either below the detection limits (chromium, copper, lead, zinc) or, if the metal was detected (cadmium, zinc), it was below the ANZECC & ARMCANZ water quality trigger concentrations for the protection of 99% of species. For these trace metals, we infer a low probability of adverse effects of sediment resuspension on pelagic biota. Note that for copper, the detection limit was below the trigger values for the protection of 95% of species. Elutriate extracts of deeper and surface sediments from three of the five sites contained nickel at concentrations that exceeded the ANZECC & ARMCANZ water quality trigger concentrations for the protection of 99% of species. The release of nickel from surface sediment likely represents a natural process because wave-driven currents suspend surface sediment. Note, however, that the concentrations of nickel AUT Client report: TTRL 20138 3 in the elutriate extracts did not exceed the ANZECC & ARMCANZ water quality trigger concentrations for the protection of 95% of species. At all sites except one, the sediment concentrations of chromium and nickel increased from the sediment surface to a depth of 5 m, the maximum depth measured. If this trend continued in deeper sediment, mobilisation of this sediment could result in seawater concentrations of chromium and nickel that exceed ANZECC & ARMCANZ water quality trigger concentrations. Additional analyses of slurries of sediment collected at one-metre increments to a maximum sediment depth of 18 m in mining areas Diana and Christina, however, did not show evidence for such a trend. We found no consistent increase with depth in the concentrations of dissolved nickel. The concentrations of chromium were below the detection limit. Processing of South Taranaki Bight sediment by steps of magnetic separation and milling increased the acid-extractable zinc and copper concentrations: the extracts from this iron sand contained two orders of magnitude higher concentrations of zinc and copper than the extracts from sediment core samples. These results were confirmed by elutriate tests. For zinc, the elutriate concentrations exceeded the ANZECC & ARMCANZ trigger values for the protection of 99% of species in three of 20 samples. For copper, the concentrations in elutriates of as-received (magnetically enriched iron sand) and coarse (first grind) iron sand exceeded the ANZECC & ARMCANZ trigger values for the protection of 99 and 95% of species. Copper concentrations in elutriates of medium and fine iron sand (second and third grind) exceeded the ANZECC & ARMCANZ trigger values for the protection of 80% of species. Iron sand particles size and elutriate copper concentrations were negatively linearly correlated. That is, the concentration of copper in iron sand elutriates increased with decreasing particle size. Assuming that South Taranaki Bight seawater contains ~0.00025 g m-3 (0.25 ppb) copper a 160-fold dilution would decrease the highest measured average copper concentration (0.0081 g m-3) to below the concentration limit for the protection of 99% of species. We recommend that TTR is taking a precautionary approach routinely monitoring seawater concentrations of copper and other trace metals in the vicinity of their beneficiation plants to ensure compliance with ANZECC & ARMCANZ guidelines. AUT Client report: TTRL 20138 4 Introduction Trans-Tasman Resources Limited (hereafter, TTR) plans to exploit a source of vanadium-titanomagnetite (VTM) in South Taranaki Bight, North Island, New Zealand, combining an offshore dredge-mining operation with an offshore beneficiation plant (Fig. 1). VTM is a black iron ore that comprises vanadium pentoxide (V2O2), titanium oxide (TiO2), and magnetite (Fe3O3), a form of iron oxide with natural magnetic properties. It originates as crystals in volcanic rocks, washed down rivers, largely from Mount Taranaki but also from the Central Plateau of the North Island, to Taranaki Bight (Carter 1980). Specialised steel mills use VTM to manufacture steel, either as a blend with other ores, e.g., haematite, or as a dedicated steel-making feed. To source iron sand from South Taranaki Bight, TTR envisages deploying seabed dredges that pump a slurry of sediment and seawater to their offshore beneficiation plant. This plant will screen the slurry to remove >2 mm particles and then extract and concentrate VTM with alternating steps of magnetic separation and grinding. TTR plans to grind iron sand particles with IsaMillTM technology to target P80’s of 150 µm (first grind) and 75 µm (second grind). Each of the processing steps onboard the beneficiation plant results in tailings and loss of seawater. In the final step, TTR will use freshwater to rinse chlorides from the concentrated iron ore. They will return the de-ored sediment to the seafloor and transfer the concentrated iron ore to a storage vessel. This ore will eventually be transhipped to a capesize vessel for export to customers. The proposed mining operation involves two activities that may result in the release of contaminants into the water column of South Taranaki Bight: (1) TTR will displace and suspend anoxic sediment from depths up to 15 m in the seabed. Suspension of this sediment may mobilise sediment-bound trace metals through oxidation of trace-metal binding solid phases (Elderfield et al. 1981, Emerson et al. 1983, Dyrssen and Kremling 1990). (2) TTR will grind the suspended sediment particles and so increase the specific surface area of the sediment. This may increase the sediment reactivity and its potential to mobilise trace metals. One mechanism for such mobilisation involves the oxidation of metal sulfides upon contact of the sediment with oxygenated seawater. Most trace metals, however, do not form distinct sulfides but adsorb onto pyrite and iron monosulfides, the most abundant solid sulfide phases in marine sediments (Huerta-Diaz and Morse 1992, Morse 1994, Gagnon et al. 1997, Huerta-Diaz et al. 1998). Oxidation of these sulfide phases upon sediment suspension may release the adsorbed trace metals into the AUT Client report: TTRL 20138 5 water column making them available for biota (Gagnon et al. 1995, Huerta-Diaz et al. 1998).
Details
-
File Typepdf
-
Upload Time-
-
Content LanguagesEnglish
-
Upload UserAnonymous/Not logged-in
-
File Pages62 Page
-
File Size-