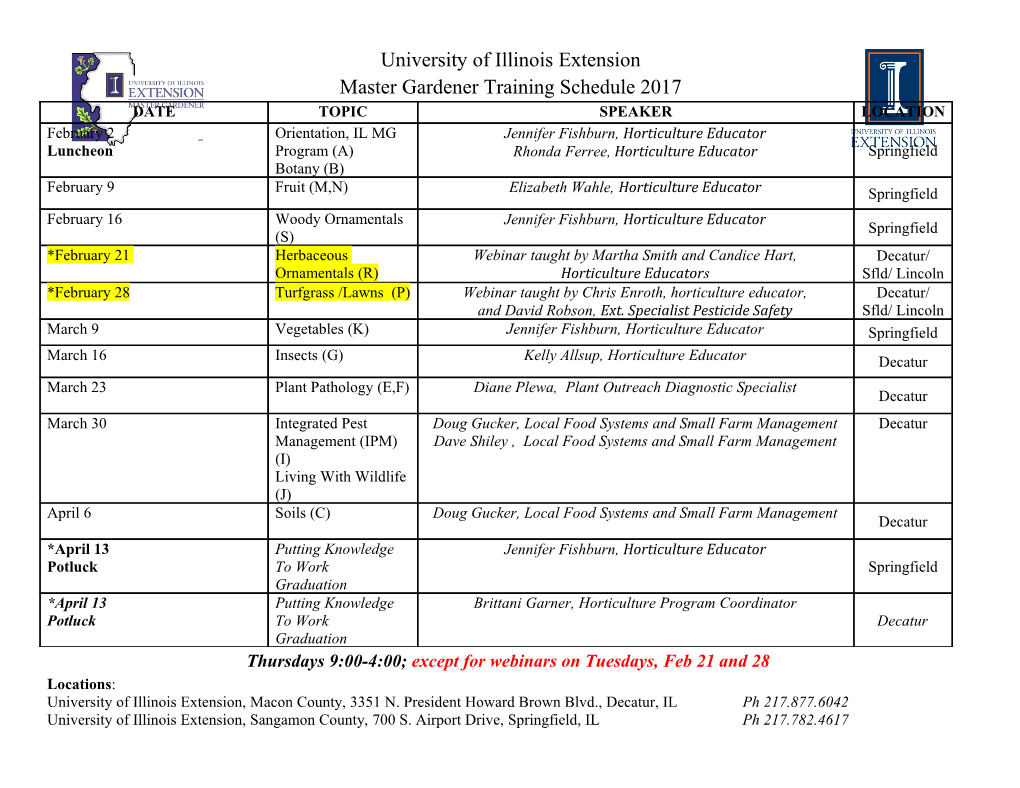
Fastnet: Early Telerobotic Exploration of the Lunar Farside by Astronauts at Earth-Moon L2 Josh Hopkins FISO Colloquium, November 14 2012 Copyright 2012 Lockheed Martin Corporation. All Rights Reserved 1 Stepping Stones Stepping Stones is a series of exploration 2023 missions building incrementally towards Deimos Scout the long term goal of exploring Mars. Each mission addresses science objectives relating to the formation of the solar 2031-2035 system and the origins of life. Red Rocks: explore Mars from Deimos 2024, 2025, 2029 2017 Plymouth Rock: Humans explore asteroids like Asteroid scout 1999 AO10 and 2000 SG344 2018-2023 Fastnet: Explore the Moon’s far side from Earth-Moon L2 point 2016 2017 Asteroid survey SLS test flight 2013-2020 Human systems tests on ISS Lockheed Martin notional concept Dates subject to change Deimos photo courtesy of NASA-JPL, University of Arizona 2 ‘Fastnet’ L2-Farside Missions • Send astronauts to the Earth-Moon Lagrange point beyond the Moon (EM-L2) • Teleoperate vehicles on the lunar farside surface • Demonstrate Orion operations in deep space with fast reentry as practice for later missions • Initial ~30 day mission into trans-lunar space using Orion only • Later flights would dock to a habitat to extend mission durations to 2-4 months 3 Rationale for Lunar Farside Exploration 4 Why The Farside? Planetary Science • To understand the impact N history of the terrestrial planets and dynamics of the early solar system we need samples of the most ancient impact basin on the farside – “The exploration and sample return from the Moon’s South Pole-Aitken (SPA) basin is among the highest priority activities for solar system science” 2011 Planetary Sciences Decadal Survey S Lunar farside elevation map courtesy NASA GSFC/DLR/ASU 5 Was the “Late Heavy Bombardment” Real? • Large lunar impact basins sampled by Apollo all appear to be 3.85-4.0 billion years old • Was there a surge in impact activity in the inner solar system at that time? • The “Nice Model” hypothesizes that rearrangement of the giant planets disrupted the entire solar system around this time • Returning samples to Earth from SPA basin can help pin down what was happening in the early solar system Impact Cataclysm? (Or Not) First signs Cambrian Explosion (complex life appears) of Life On Earth Impact Flux Impact 4.5 4.0 3.0 2.0 1.0 Today Time, Billions of Years Ago 6 Why The Farside? Cosmology • The lunar farside is the only radio-quiet zone where we can observe low frequency signals from the earliest formation of stars and galaxies – “A great mystery now confronts us: When and how did the first galaxies form out of cold clumps of hydrogen gas and start to shine—when was our ‘cosmic dawn’?” 2010 Astrophysics Decadal Survey • Signals from neutral hydrogen at 21 cm wavelength (1420 MHz) during this early time period have been redshifted by a factor of 10-100 down to frequencies of 10s of MHz – Artificial radio signals and natural noise from the ionosphere interfere with this faint signal on Earth and in orbit 7 Radio Astronomy Instrument • A lunar rover could unroll antennas printed on polyimide film directly onto the lunar surface – Scale is hundreds of meters to few km due to long wavelength – Antenna hardware is in early development phase – In 2013 astronauts on ISS will attempt to teleoperate rover deployment of an antenna at NASA Ames • An initial flight could unroll a single shorter arm prototype for test purposes • Later mission would unroll three arms for full resolution • Operational instrument will need communications relay from the farside at ~10 Mbs data rate 8 How Do Astronauts Help? • Launching samples up to Orion instead of directly back to Earth may enable returning much larger sample mass from the same size lunar lander • Astronauts at EM-L2 can teleoperate a rover on the lunar farside more effectively than from Earth due to reduced speed of light latency – Approx 0.4 sec from L2 vs 3.5 sec from Earth via L2 relay (plus network latency) • The Moon is a good place to practice orbital teleoperation of rovers on a planetary surface before we try to do the same thing from Mars orbit – Deimos to Mars two-way speed of light latency is ~0.15 sec 9 Payoff from Reduced Latency • We tested multiple users on a 3.5 lunar driving simulator using Average of all drivers varying latency 3.0 • Driving course with 7 waypoints over ~100 m 2.5 distance, rough topography, 2.0 and 0.6 m/s maximum rover speed 1.5 • Minimal dexterity/precision required, no rover autonomy 1.0 • Test results suggest a factor 0.5 of 2 improvement in speed operating from L2 (~0.5 sec) duration,Driving normalizedto 1= no latency 0.0 0 1 2 3 4 5 6 instead of Earth (4 to 5 sec) Added control latency, seconds 10 Screen capture from lunar rover simulator 11 Value for Exploration • Astronauts at L2 can contribute to farside science, but not enough to justify the cost of a human mission on science grounds alone • L2 also provides an important proving ground for human missions to deep space such as asteroids, Mars – It allows us to “dip our toes in” deep space on short missions – Learning to do human spaceflight beyond the Earth’s magnetosphere – At the end of a much longer logistics chain than ISS – Learning to operate safely when abort return is possible, but not fast 12 L2/Farside Mission Design 13 Types of Orbits Near L2 • If a spacecraft is offset from the L2 (or L1) point, it oscillates independently in Y and Z along a Lissajous curve (examples in Blue) +Z • We can pick the Y and Z amplitudes to synchronize the periods in both axes, creating a +Y halo orbit (examples in Red) • Large amplitudes are not good 20,000 km for providing continuous comm to the lunar surface • The smallest halos have Y amplitudes > 32,000 km • Lissajous orbits can have smaller amplitudes, but pass behind the Moon as seen from Earth (communications problem) View looking towards the Moon from Earth 14 L2 Halo & Lissajous Orbit Geometry Top View (Looking “down” on the Moon’s orbit plane) L1 L2 GEO Earth Moon Side View (Parallel to Moon’s orbit plane) 15 Trajectory Options to L2 Region • For crew: Lunar flyby to L2, ~ 9-12 days, 250-325 m/s ΔV • For outpost deployment & resupply: Ballistic lunar transfer, ~95 day, < 10 m/s ΔV (data for one-way trip) Mid-Course Maneuver L2 Moon Direct Flyby Solar Vector Earth Earth Orbit of L2 Lunar 100,000 km Orbit Inertial Reference Frame 16 Example Reference Trajectory Early mission of Orion only, before an outpost habitat is available at L2 Trans-Lunar Injection (TLI) Reentry, 11 km/s Cruise to Moon, 5 Days Earth return cruise, 5 Days L1 Outbound Perilune Return Lunar Flyby and Retro Burn, 185 m/s Retro Burn, 176 m/s C L2 A Earth-Moon Rotating Reference Frame D Nominal Mission Duration: 33 Days B Duration Beyond the Moon: 22 Days A: Halo Targeting Burn: 58 m/s Nominal Orion ΔV ~470 m/s B: Halo Insertion Burn: 13 m/s Longest Eclipse ~54 min C: Halo Departure: <1 m/s Only one communications gap of 2.5 hours D: Pre-perilune Burn : 24 m/s 17 Spacecraft Configurations 18 Orion • Orion is already being designed for lunar missions and the lunar environment Launch Abort • Open space is generally more benign System (LAS) than low lunar orbit • No major redesign is expected to perform missions to L2 region Crew Module • Two upgrades may be of interest (CM) – Accommodating secondary payloads Service Module (SM) – Extending mission duration Adapters 19 Potential Secondary Payload Locations Forward Compartment Dimensions are approximate, 26” (If no docking mechanism) pending clearance analysis 660 mm 30” 760 mm 68” 42” 1725 mm 1065 mm SIM Bay (Standard) Aft Compartment (If no main engine) 40” 1015 mm 68” 144” 1725 mm 3660 mm 21” 530 mm 20 Forward Compartment Option Launch Abort System Truss Assembly Representative Spacecraft Payload Interface Ring Crew Module Fwd Bay 21 Extended Mission Duration • Baseline Orion crew mission capability is 21 days, limited primarily by life support consumables • Water, O2 and N2 supply can be extended to >30 days by changing ECLSS tank size GO2 tanks (2) GN2 tanks (2) Water tanks (5) Baseline 21 Day Crew Capability Extended Duration Capability 22 Added Pressurized Stowage • Mission duration is limited by stowage volume and mass limits in the Crew Module for food, waste tanks, etc. • This could be augmented by a “pantry module” on the forward docking adapter which jettisons prior to reentry. – Could stow supplies for up to 60 day missions – But, would preclude docking to other vehicles (e.g. habitat) Medium Pantry Module Total Pressurized Volume: 85 ft3 (2.4 m3) Cargo volume: 58 ft3 (1.8 m3) 23 International Industry Collaboration • International industry team formed in 2009 to study next steps in human exploration, focusing on an L2 outpost supporting lunar farside missions • Team members are prime contractors from most of the ISS participating countries • Jointly investigating ways to apply heritage systems to L2 outpost and robotic lunar surface functions 24 Why an Outpost at EM-L2? • Our companies and agencies have different priorities. An EM-L2 outpost is potentially compatible with multiple objectives such as – Relay and control node for lunar surface robotics – Preparing for missions into deep space (radiation protection, psychological health, crew autonomy) – Possible transportation hub for human lunar landings or missions to asteroids or Mars – Servicing space telescopes • EM-L2 is easier to reach than lunar orbit, GEO, or EM-L1 so multiple countries can contribute using their ISS- derived capabilities 25 Rendezvous in Halo Orbits • Rendezvous with a pre-deployed habitat in the halo orbit imposes an additional launch window constraint – Budget of ~100 m/s buys launch opportunities on 4 consecutive days every 14-15 days – Budget of ~300 m/s allows launch on any date, but with trip times that vary by several days • Halo orbits are in a shallow gravity field with long periods, so rendezvous is not like LEO operations – No phasing orbits.
Details
-
File Typepdf
-
Upload Time-
-
Content LanguagesEnglish
-
Upload UserAnonymous/Not logged-in
-
File Pages29 Page
-
File Size-