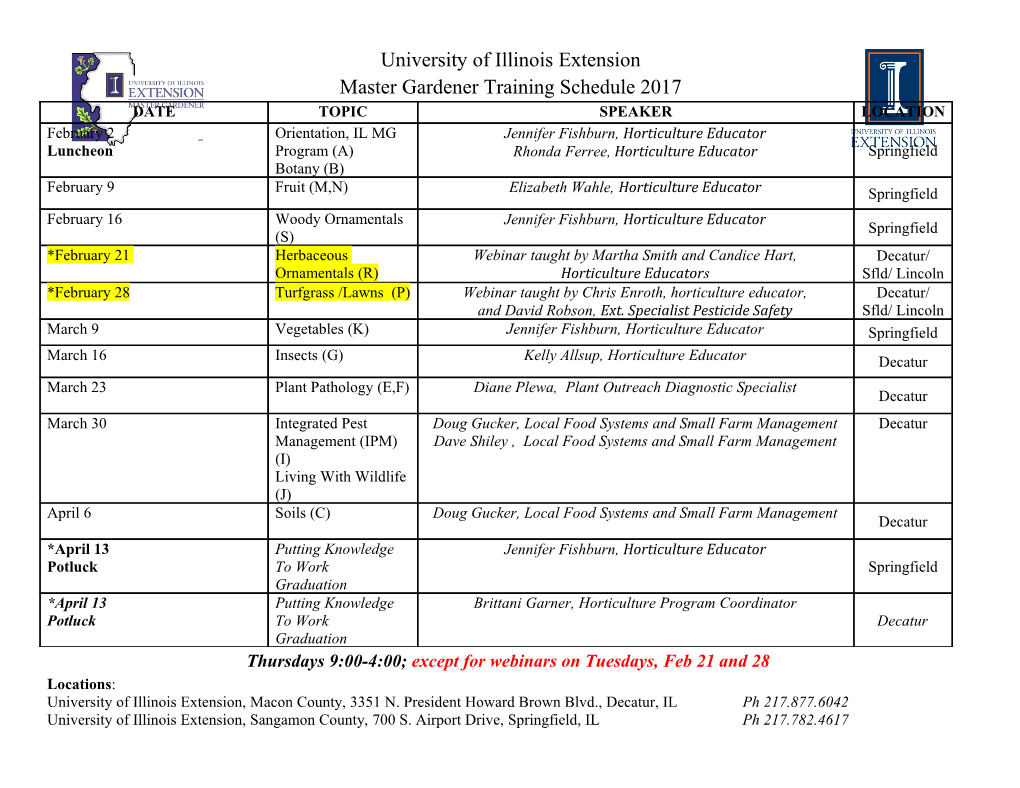
CRANFIELD UNIVERSITY Samer Aldhaher Design and Optimization of Switched-Mode Circuits for Inductive Links School of Engineering Energy & Power Engineering Division PhD Thesis Academic Year: 2010 - 2014 Supervisor: Prof. Patrick Chi-Kwong Luk January 2014 ii CRANFIELD UNIVERSITY School of Engineering Energy & Power Engineering Division PhD THESIS Academic Year 2010 - 2014 Samer Aldhaher DESIGN AND OPTIMIZATION OF SWITCHED-MODE CIRCUITS FOR INDUCTIVE LINKS Supervisor: Prof. Patrick Chi-Kwong Luk January 2014 This thesis is submitted in partial fulfilment of the requirements for the degree of Doctor of Philosophy © Cranfield University 2014. All rights reserved. No part of this publication may be reproduced without the written permission of the copyright owner. iv to my parents vi Abstract Wireless power transfer (WPT) via magnetic induction is an emerging technology that is a result of the significant advancements in power electronics. Mobiles phones can now be charged wirelessly by placing them on a charging surface. Electric vehicles can charge their batteries while being parked over a certain charging spot. The possible applications of this technology are vast and the potential it has to revolutionise and change the way that we use today’s application is huge. Wireless power transfer via magnetic induction, also referred to as inductive power transfer (IPT), does not necessarily aim to replace the cable. It is intended to coexist and operate in conjunction with the cable. Although significant progress has been achieved, it is still far from reaching this aim since many obstacles and design chal- lenges still need to be addressed. Low power efficiencies and limited transfer range are the two main issues for IPT. A tradeoff is usually associated with these two issues. Higher efficiencies are only achieved at very short transmission distances, whereas transferring large amounts of power at large distances is possible but at reduced effi- ciencies. This thesis addressed the limitations and design challenges in IPT systems such as low efficiency and short transmission range, in addition to poor power regulation and coil displacement and misalignment sensitivity. Novel circuit topologies and design solutions have developed for DC/AC inverters and DC/AC rectifiers that will allow for increased performance, higher efficiencies and reduced sensitivity to coil misalign- ments and displacements. This thesis contributes in four key areas towards IPT. Firstly, a detailed mathematical analysis has been performed on the electric circuit model of inductively coupled coils. This allows for better understanding on how power is distributed amongst the circuit’s elements. Equivalent circuit representations were presented to simplify the design process of IPT systems. Secondly, a review of the different classes and configurations of DC/AC inverters that can be used as primary coil drivers in IPT systems were presented. Class E DC/AC inverters were mathematically analysed in great detail and their performance as primary coil drivers in IPT systems was investigated. Thirdly, novel electronic tuning methods were presented to allow Class E primary coil drivers to operate at optimum switching conditions regardless of the distance between the coils of an IPT system and the value of the load. The saturable reactor was used as the electronic tunable element. Lastly, Class D and Class E AC/DC rectifiers have been used for the first time in IPT systems. Detailed mathematical analysis and extensive experimental results show their superior performance over the conventional half-wave and full-wave AC/DC rectifiers. viii Acknowledgements I would like to thank my supervisor Professor Patrick Luk for his supervision and experienced guidance. His continuous support and encouragements gave me more confidence in my work and abilities and led me to complete my project successfully. I would also like to thank my second supervisor Dr. James Whidborne for his valuable discussions, cooperation and support. This journey would not have been pleasant without my friends and fellow PhD students at Cranfield University, especially Milena Traczyk, Francis Salama, Quen- tain McEnteggert, Robert Christie, Kirsty Wallis, Sue Richardson, Atma Parkash and Solange Baena. I am very grateful to Glenda Metcalf for her continuous support, love and friendship since day one. I owe every part of this work, my education and every step forward in my life to my parents. I would like to thank them for their love, support and encouragement. x xi CONTENTS 1 introduction1 1.1 Overview of an Inductive Power Transfer System . 1 1.2 Motivation . 3 1.3 Aims and Objectives . 3 1.4 Methodology . 4 1.5 Thesis Structure . 4 1.6 Contributions . 6 1.7 Publications . 7 2 wireless power transfer technology9 2.1 Introduction . 9 2.2 Historical Achievements and Scientific Breakthroughs . 10 2.3 Wireless Power Transfer Methods . 14 2.3.1 Capacitive Coupling . 14 2.3.2 Laser Coupling . 15 2.3.3 Microwave Coupling . 16 2.3.4 Pressure Waves . 19 2.4 Related Work from the Literature in WPT via Magnetic Induction (IPT) 20 2.4.1 Current Applications and Technologies . 20 2.4.2 Research Trends . 30 2.5 Suggested applications based on IPT . 34 2.6 Conclusions . 39 3 inductive links 41 3.1 Introduction . 41 3.2 Modelling of Inductive Links . 41 3.2.1 Quantities and Parameters . 41 3.2.2 Equivalent Series Resistance and Quality Factor . 45 3.2.3 Circuit Representation . 47 3.3 Resonant Coupling . 50 3.4 Analysis of a Parallel Resonant Secondary Coil . 52 3.4.1 Near Resonance Operation . 53 3.4.2 Reflected Load Formulae . 53 3.4.3 Load and Frequency Variation Effects On The Reflected Impedances 58 3.5 Conclusions . 61 xii CONTENTS 4 resonant dc/ac inverters 63 4.1 Introduction . 63 4.2 Definitions . 64 4.3 Class D Inverters . 66 4.3.1 Half-Bridge Class D ZVS Inverter . 66 4.3.2 Class D Push-pull ZVS Inverter . 72 4.3.3 H-Bridge Class D ZVS Inverter . 73 4.3.4 Class D ZCS Inverter . 75 4.3.5 Class D Push-Pull ZCS Inverter . 77 4.4 Class E Inverters . 79 4.4.1 The Class E ZVS and ZVDS Inverter . 80 4.4.2 Class E push-pull ZVS and ZVDS Inverter . 87 4.4.3 Class E Inverter with Finite DC-feed Inductance . 87 4.4.4 State-space Modelling . 95 4.4.5 Sub-optimum Operation . 100 4.5 Other Classes and Configurations . 104 4.5.1 Class E Current-switching Inverter . 105 4.5.2 Class DE Inverters . 106 4.6 Conclusions . 107 5 design and optimization of class e primary coil drivers 109 5.1 Introduction . 109 5.2 Modelling and Analysis of an Inductive Link with a Class E Driver . 109 5.2.1 Design . 109 5.2.2 Analysis Using the Analytical Approach . 110 5.2.3 Analysis Using the State-space Representation Approach . 113 5.2.4 Design Example . 116 5.2.5 Performance Investigation . 117 5.2.6 Developing Tuning Methods and Requirements . 119 5.3 Saturable Reactors as Electronic Tuning Elements . 120 5.3.1 Magnetic Cores . 121 5.3.2 Design Trade-offs . 122 5.4 Tuning The Class E Inverter with Infinite DC-feed Inductance . 123 5.4.1 Frequency and C1 control . 123 5.4.2 Experimental Verification . 124 5.4.3 Implementation of the proposed tuning method . 126 5.5 Tuning The Class E Inverter With Finite DC-feed Inductance . 129 5.5.1 Frequency and Lf control . 130 5.5.2 Optimisation . 133 5.6 Tuning Methods Based on State-Space Modelling . 138 5.6.1 Duty cycle and Lf control . 139 CONTENTS xiii 5.6.2 Simulink modelling . 139 5.6.3 Implementation of the proposed tuning method . 140 5.7 Conclusions . 142 6 ac/dc rectifiers in ipt systems 145 6.1 Introduction . 145 6.1.1 Definitions . 146 6.2 Traditional Rectifiers . 148 6.2.1 Half-Wave Rectifiers . 148 6.2.2 Full-Wave Rectifiers . 149 6.2.3 Performance Investigation . 150 6.3 Class D Rectifiers . 152 6.3.1 Analysis of Class D Voltage-driven Rectifiers . 153 6.3.2 Analysis of Class D Current-driven Rectifiers . 156 6.3.3 Performance Investigation of Class D Voltage-driven Rectifiers . 158 6.4 Class E Rectifiers . 170 6.4.1 Principle of Operation . 172 6.4.2 Modelling and Analysis of a Class E ZVS, Low dv=dt Rectifier . 175 6.4.3 Input Current linearity and Harmonic Content . 180 6.4.4 Experimental Setup and Results . 183 6.5 Conclusions . 186 7 class e2 resonant dc/dc converters 189 7.1 Introduction . 189 7.2 Modelling and Analysis . 190 7.2.1 State-space Representation . 190 7.2.2 Switching Periods and Operating Modes . 192 7.2.3 Determining the Initial Conditions of the States . 193 7.2.4 Solving for Optimum Switching Conditions . 194 7.3 Design Case . 195 7.3.1 Initial Design . 195 7.3.2 Comparison with the Analytical Approach . 198 7.3.3 Compensation for Load Variations and Coil Misalignments . 200 7.4 Experimental Verification . 202 7.4.1 Implementation and Set-up . 202 7.4.2 Class E Rectifier Self-start and Driving circuit . 202 7.4.3 Results . 202 7.4.4 Load Variation . ..
Details
-
File Typepdf
-
Upload Time-
-
Content LanguagesEnglish
-
Upload UserAnonymous/Not logged-in
-
File Pages306 Page
-
File Size-