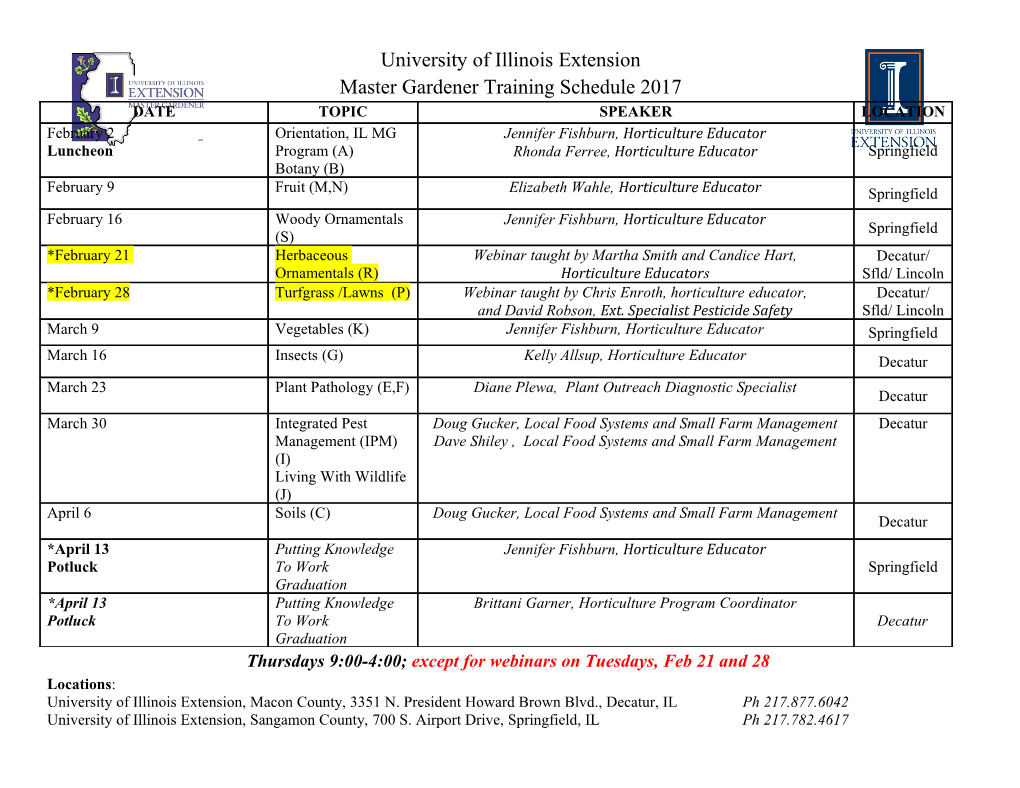
Trans. JSASS Space Tech. Japan Vol. 7, No. ists26, pp. Pb_59-Pb_63, 2009 Comparative Investigation of Fusion Reactions for Space Propulsion Applica- tions By Dejan PETKOW, Georg HERDRICH, Rene LAUFER, Roland GABRIELLI and Oliver ZEILE Institute of Space Systems, Universitaet Stuttgart, Germany (Received May 2nd, 2008) A space propulsion system based on the acceleration of fusion ash is discussed by use of the energy balance equation and a hypothetic ash extraction and acceleration system. The fusion reactions D-T, D-3He, p-11 B and 3He-3He are investi- gated under the condition of thermal generation of high energy ions and equal plasma system conditions in terms of T i/T e relation and plasma beta. External plasma heating is defined by an equal efficiency concerning thermal energy conversion and energy transfer back into the plasma. There is no additional external heating applied to the fusion system. Power losses are based on neutrons, bremsstrahlung, synchrotron radiation and convection. We compare the plasma pressures, volumet- ric power densities, magnetic field strengths, heat waste, exhaust velocities and thrust density levels depending on the tem- perature and the hot ion mode. We show that, based on the fusion products, the exhaust velocity may reach several percent of speed of light in the case of 3He-3He. The temperature driven radiation losses of the 3He-3He reaction puts the purely aneutronic property into perspective. The mass flow rate densities of the considered fusion products are very low leading to very low thrust power densities. Considering the supposed system masses of a fusion based space vessel the thrust density levels are negligible and reach the order of 1 N/m 3 near the optimum in the case of 3He-3He. We conclude that a propulsion system based on the acceleration of fusion products or ash is unfeasible for typical manned missions e.g. to Mars. Key Words: Space Propulsion, Fusion, Aneutronic, Plasma Nomenclature Subscripts F :fusion D :deuterium (-) m :magnetic T :tritium (-) th :thermal p :proton (-) Br :bremsstrahlung 11 :boron-11 (-) B Sy :synchrotron radiation 3He :helium-3 (-) N :neutron 3 P :power density (W/m ) e :electron p :pressure (Pa) i :ion species E :energy (J) j :species counter 2 σ :cross section (barn, m ) k :power source T :temperature (keV) Superscripts :ion temperature (keV) ´ :temperature in K Ti v :velocity (m/s) 1. Introduction kB :Boltzmann constant (J/K) Nuclear fusion is investigated as a future option for M : effective mole mass (kg/mol) eff commercial energy production 1-3) and discussed as a fu- n -3 :particle density (m ) ture option for space propulsion purposes 4-6). The diffi- :Avogadro constant (mol -1) N A culties in realizing a fusion based propulsion system are Q :power factor (-) fundamental as they are in case of terrestrial fusion reac- tors: fusion plasmas burn at extremely high temperatures S :constant (~) and one of the core problems is to achieve these tempera- Z :charge number (-) tures. Other problems concern e.g. the high temperature B :magnetic field (T) resistivity of the reactor material and the generation of c* :constant (1/keV) very high magnetic field strength for the plasma confine- η :energy absorption coefficient for reactor wall(-) ment. :relation of product ion energy to total Besides that, fusion based energy production on Earth ζ fusion energy release (-) demands technologies which are quite different from ϕ :hot ion mode temperature relation (-) those for space applications in terms of complexity, reli- δik :Kronecker symbol (-) ability, redundancy, accessibility, mass, heat and cost as- 7) µ :vacuum permeability (Vs/Am) pects . The first three aspects are strongly connected to 0 each other leading to the statement that the space propul- Copyright© 2009 by the Japan Society for Aeronautical and Space Sciences and ISTS. All rights reserved. Pb_59 Trans. JSASS Space Tech. Japan Vol. 7, No. ists26 (2009) sion system has to be as simple as possible. The D-T reac- size. It is assumed that a hypothetical mechanism exists tion is usually disregarded due to the intense production which perfectly extracts and accelerates the fusion ash of neutrons which heat up the reactor wall. Since moving after its thermalization. Wall erosion effects are ne- parts e.g. for calorimetric energy conversion are to be glected. avoided as far as possible and heat waste can be removed only by large and heavy radiators, heat losses to the wall 2.1. Propellant data have to be minimized. Correspondingly, this leads to the We discuss the four most popular (neutronic as well as common conclusion that aneutronic fusion reactions are aneutronic) fusion propellants: the most promising candidates. However, it is well known 1. D + T 4He + n + 17.6MeV that the aneutronic 3He-3He reaction needs extremely high 2. D + 3He 4He + p + 18.3MeV temperatures for ignition and operation which is accom- 3. p + 11 B 3 4He + 8.7MeV panied by strong radiation losses of just another type 4. 3He + 3He 4He +2p + 12.9MeV (bremsstrahlung) but with the same wall heating effect. As For the sake of simplicity, side reactions as e.g. the a consequence, it is unknown which fusion propellant is D-D reactions are neglected and the fusion plasma is con- more feasible for space propulsion. It is the objective of sidered as homogeneous. The fusion rates base on the re- this work to compare of the four most popular fusion pro- action rate coefficients <σv> which are generally ob- pellants which are usually discussed separately and not on tained by averaging the fusion cross section over an equi- a system level. librium energy distribution. The structure and proceeding of this study is as follows: 1E+01 In section 2 the theoretical basics are briefly introduced. After introducing system based model, the power loss and 1E+00 gain terms are briefly introduced and balanced by apply- 1E+01 1E+02 1E+03 1E+04 1E+05 ing system parameters (efficiencies) which are equal for all propellants. The balance equation leads to an extended 1E-01 burn criterion expressed by the product of ion particle barn / σ σ σ σ density ni times the energy confinement time τE. Results like volumetric power densities, mass flow rate densities, 1E-02 D-T D-He3 exhaust velocities and thrust density levels for different p-11B fuel ashes are discussed in section 3. The key aspects of 3He-3He 1E-03 this investigation are summarized and conclusions are E / keV made in section 4. 11, 12) Fig. 2. Fusion cross section data. 2. Theoretical Considerations Hence, it is important to know the fundamental data – the cross sections, see Fig. 2. The numerically integrated Generally, a space vessel consists of many systems, reaction rate constants are depicted in Fig. 3. subsystems etc. Within the scope of this work we consider fusion plasma, reactor wall, energy conversion, heat trans- port, radiator and energy recirculation as separate subsys- tems or system properties which are connected by power fluxes. The simplified model is depicted in Fig. 1. Fig. 3. Reaction rate constants. 2.1. Energy balance Fig. 1. Simplified model of the fusion propulsion system In order to make a comparison between the discussed The subsystem fusion plasma gains power from the fusion propellants we use the energy balance equation. fusion reactions and from recirculated power but looses Radiative losses are given 8 by: power to the wall. Outside the reactor wall energy con- P S T n n Z 2 version devices are assumed converting heat to electric Br = Br e e ∑( j j ) (1) j power. Unconvertable heat waste is transported to the and radiators. 2 * The fusion reactor in this study is based on a closed PSy = SSy B neTe 1( + c Te ) . (2) magnetic plasma confinement and has no defined shape or The neutron power is defined as: Pb_60 D. PETKOW et al.: Comparative Investigation of Fusion Reactions for Space Propulsion Applications PN = 1( − ζ )PF . (3) strengths for the confinement following Eq. 7. The results Thermal losses are described as follows: are depicted in Fig. 5. The D-T reaction demands the low- est magnetic field strengths (1-10 T) which represents in Pth = Eth /τ E . (4) the area around the optimum point available technology. Neutrons, bremsstrahlung and thermal losses are fully For 3He-3He the magnetic fields are very advanced and in absorbed by the wall as well as 10 % of the synchrotron the range of approximately 300 T. radiation. It is assumed that 30 % of this heat wall load is Figure 6 shows the plasma power density for all propel- converted into electric energy and coupled back into the lants and for different hot ion modes. The D-T plasma has plasma leading to a sort of external heating P . The sec- ext the lowest plasma density (1-10 MW/m 3). The power den- ond gain term is represented by: sity of 3He-3He is four orders of magnitude higher than 3 ni nk that (10-100 GW/m ). PF = ζ < σv > EF (5) 1 + δ ik 1 + δ ik 1E+08 3He- 3He Due to ζ the neutron losses affect only Pext since they 1E+07 cannot be confined by the magnetic field and are therefore 1E+06 p- 11 B D-T not explicitly treated as a loss in the balance equation. D- 3He Introducing the hot ion mode one can substitute the elec- 1E+05 1E+04 tron temperature using the relation Ti/T e = φ. A hot ion / bar th φ=1 mode is characterized by φ > 1 and reduces the losses via p 1E+03 φ=2 15, 16) bremsstrahlung which is a well known effect .
Details
-
File Typepdf
-
Upload Time-
-
Content LanguagesEnglish
-
Upload UserAnonymous/Not logged-in
-
File Pages5 Page
-
File Size-