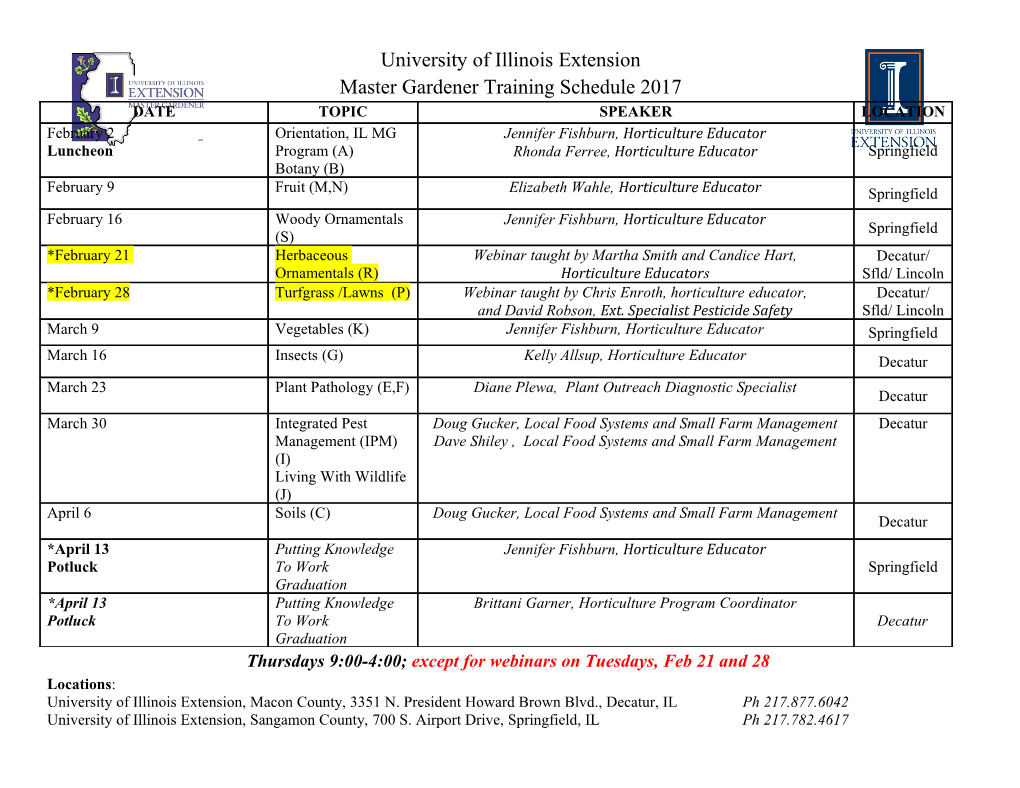
CHAPTER THREE Epigenetics: The Second Genetic Code Nathan M. Springer* and Shawn M. Kaeppler† Contents 1. Introduction 60 2. Molecular Mechanisms of Epigenetic Inheritance 60 2.1. DNA methylation 61 2.2. Histone modifications 62 2.3. Chromatin structure 63 2.4. Role of RNA in heritable silencing 64 2.5. Interactions among DNA methylation, histone modifications, and chromatin structure 65 3. Epigenetic Phenomena in Plants 65 3.1. Phenotypic examples of epigenetic inheritance 66 3.2. Genomic and molecular genetic examples of epigenetic variation 70 4. Epigenetic Inheritance and Crop Improvement 71 4.1. Epigenetics in quantitative inheritance and selection response 72 4.2. Epialleles and gene discovery 73 References 73 Abstract Plant breeders utilize directed selection and transgenics to produce novel cultivars of diploid and polyploid species. DNA sequence is clearly important in these processes, but growing evidence implicates epigenetics as an impor- tant factor in controlling genetic variation and gene/transgene expression. In this article, we focus on epigenetic variation defined as mitotically and meioti- cally heritable but reversible states of gene expression that are not conditioned by differences in DNA sequence. We summarize mechanisms underlying epige- netic states of expression, and discuss implications of epigenetics in cultivar development. * Department of Plant Biology, University of Minnesota, Saint Paul, Minnesota 55108, USA { Department of Agronomy, University of Wisconsin, Madison, Wisconsin 53706, USA Advances in Agronomy, Volume 100 # 2008 Elsevier Inc. ISSN 0065-2113, DOI: 10.1016/S0065-2113(08)00603-2 All rights reserved. 59 60 Nathan M. Springer and Shawn M. Kaeppler 1. Introduction Plant breeders have made tremendous progress towards altering plant phenotypes to provide more productive crops. The majority of these gains have been made through selection of variation that is present within a species. While it is clear that phenotypic selection has resulted in improved plant characteristics, the molecular basis of the selected variation remains largely unknown. Traditionally, it was assumed that the majority of herita- ble variation was due to genetic sequence differences. However, a growing body of evidence suggests that intraspecific expression differences among genotypes can also be caused by epigenetic variation. To some researchers, epigenetics is used as a catchall term to describe any variation that does not seem to follow Mendelian inheritance patterns. In this article, we use the term epigenetics to specifically describe meiotically or mitotically heritable differences in gene expression that are not caused by sequence differences. We will begin by describing some of the molecular changes that are associated with epigenetic variation and then proceed to discussing some of the well characterized examples of epigenetic variation. Finally, we will discuss the potential impact of epigenetic inheritance in crop improvement. 2. Molecular Mechanisms of Epigenetic Inheritance Epigenetics involves changes in heritable phenotypes without changes in DNA sequence. With the exception of protein-based inheritance such as prions, epigenetic differences are the result of altered gene expression levels. Research on the molecular mechanisms of epigenetic inheritance has iden- tified several complementary pathways for the stable regulation of gene expression without sequence changes. The basis of epigenetic inheritance is the manner in which DNA is packaged and modified. DNA in plant cells contains four standard bases, cytosine, guanine, thymine, and adenine, but can also contain the modified base 5-methylcytosine. Most often, presence of 5-methylcytosine is asso- ciated with repressed gene expression. DNA in the cell is packaged by various sets of proteins. The first level of packaging involves wrapping DNA around cores of histone octamers containing two each of the proteins: Histone 2A (H2A), Histone 2B (H2B), Histone 3 (H3), and Histone 4 (H4). The histone proteins in the octamer can contain various modifications. The most relevant modifications for epigenetic expression are acetylation and methylation, which occur on the tails of H3 and H4. The tails of these histones extend outside of the DNA/protein core, and the modifications affect how those cores are packaged into a higher order structure. Epigenetics: The Second Genetic Code 61 Various proteins are involved in methylation of the DNA, modification of the histones, recognition of chromatin state, and in energy-dependent remodeling of one chromatin state to another. Following is a brief summary of some of the most important players in this process, chosen largely because one or more members have proven effects on gene expression in plants. 2.1. DNA methylation DNA methylation is found in the genomes of many plant and animal species. In eukaryotic genomes, DNA methylation refers primarily to 5-methylcytosine although some evidence for methylated adenines has been reported. The methyl moiety is added to cytosine residues present in double stranded DNA by a group of enzymes referred to as DNA methyl- transferases. The majority of DNA methylation is found in CpG dinucleo- tide (plants and animals) and CpHpG trinucleotide (plants only) sequence contexts. Plant genomes encode several different DNA methyltransferase enzymes that fall into three different functional categories (reviewed by Chan et al., 2005). The Domains Rearranged Methyltransferases (DRM) encode de novo methyltransferases. These enzymes are capable of adding methyl groups to DNA that is unmethylated. The other two categories, DNA methyltransferases (MET) and chromomethylases (CMT), encode maintenance methyltransferases. Following DNA replication, the parent strand retains 5-methylcytosine but all cytosines within the daughter strand are unmethylated. This hemimethylated substrate is the target of the main- tenance methyltransferases. Hemimethylated CpGs are the target of the MET class of enzymes while hemimethylated CpHpGs are methylated by CMTs. The targeting of a DRM protein to a specific genomic location will result in methylation of all cytosines within the region. If the targeting signal is no longer present then only the CpG and CpHpG methylation will be maintained by maintenance activities. These are likely oversimplifications of the activities and preferences for these enzymes as there appears to be some redundancy and locus-specific activities for these classes (reviewed by Chan et al., 2005). In general, DNA methylation is associated with transcriptional silencing of a locus. DNA methylation can result in silencing by directly interfering with the binding of transcriptional activators or by recruiting proteins that bind to methylated DNA and recruit transcriptional repressors (Bird and Wolffe, 1999; Klose and Bird, 2006). DNA methylation is often associated with centromeres and repetitive elements (Zhang et al., 2006). Recently, the application of microarrays and high-throughput sequencing approaches have provided a view of genome-wide DNA methylation patterns in Arabi- dopsis and the relationship of DNA methylation and gene expression (Cokus et al., 2008; Lister et al., 2008; Zhang et al., 2006; Zilberman et al., 2007). DNA methylation is quite high in transposon sequences and loss of CpG 62 Nathan M. Springer and Shawn M. Kaeppler methylation often results in transcriptional activation of these sequences. Two different types of genic methylation were noted (Lister et al., 2008; Zhang et al., 2006; Zilberman et al., 2007). A significant proportion of genes (33%) exhibit methylation within the coding region and a much smaller proportion of genes (5%) exhibit promoter methylation. However, a relatively small number of genes (500) exhibit altered expression when DNA methylation is reduced (Zhang et al., 2006). The majority of genes which are sensitive to DNA methylation exhibit promoter methylation. Interestingly, most of the genes controlled by CpG methylation are pseudogenes located within peri- centromeric heterochromatin while the genes regulated by CpHpG methyla- tion are spread throughout euchromatic portions of the genome (Zhang et al., 2006). There is also evidence for altered expression of antisense and nc RNAs in plants with reduced DNA methylation levels suggesting that DNA methyl- ation may be required to reduce transcriptional ‘‘noise.’’ 2.2. Histone modifications The histone proteins exhibit remarkable sequence conservation in eukaryotes within the globular head domain that interacts with other histones and DNA as well as within the ‘‘tail’’ domain that protrudes from the central octomer– DNA complex. Recent research has identified a number of posttranslational modifications that occur to the histone tails including acetylation, methylation, SUMOlation, ubiquitination, and others (reviewed by Kouzarides, 2007; Pfluger and Wagner, 2007). These histone modifications can provide a variety of functions including transcriptional activation, transcriptional repression, efficient assembly into chromatin, and DNA replication. The theory of a histone code, in which each modification indicates a specific meaning and the combinations of modifications result in interpretations of chromatin state, was proposed ( Jenuwein and Allis, 2001). However, most current research suggests that there are actually a limited number of chromatin states and that the many of the modifications can act in a redundant manner (Kouzarides, 2007; Peterson and Laniel, 2004). Histone modifications can have
Details
-
File Typepdf
-
Upload Time-
-
Content LanguagesEnglish
-
Upload UserAnonymous/Not logged-in
-
File Pages22 Page
-
File Size-