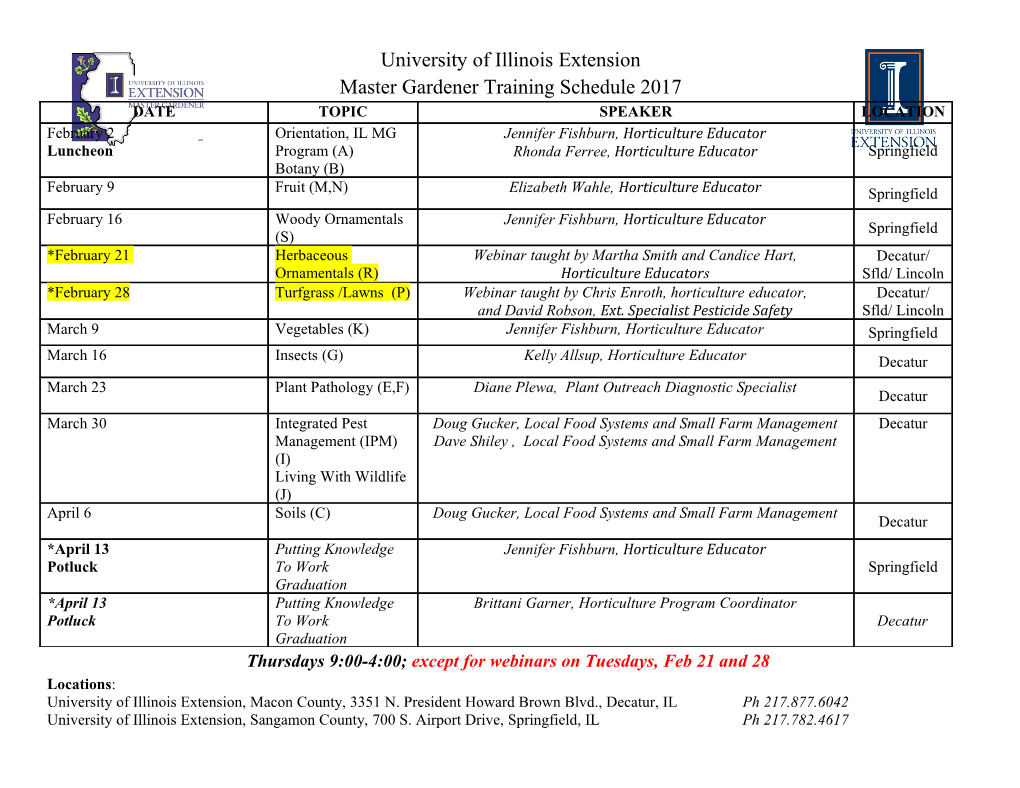
7220 J. Am. Chem. Soc. 2001, 123, 7220-7227 Mechanism of â-Hydrogen Elimination from Square Planar Iridium(I) Alkoxide Complexes with Labile Dative Ligands Jing Zhao, Heather Hesslink, and John F. Hartwig* Contribution from the Department of Chemistry, Yale UniVersity, P.O. Box 208107, New HaVen, Connecticut 06520-8017 ReceiVed February 16, 2001 Abstract: Mechanistic studies were conducted on â-hydrogen elimination from complexes of the general formula [Ir(CO)(PPh3)2(OR)], which are square planar alkoxo complexes with labile ligands. The dependence of rate, isotope effect, and alkoxide racemization on phosphine concentration revealed unusually detailed information on the reaction pathway. The alkoxo complexes were remarkably stable, including those with a variety of electronically and sterically distinct groups at the â-carbon. These complexes were much more stable than the corresponding alkyl complexes. Thermolysis of these complexes in the presence of PPh3 yielded the iridium hydride [Ir(CO)(PPh3)3H] and the corresponding aldehyde or ketone with rate constants that were affected little by the groups at the â-carbon. The reactions were first order in iridium complexes. At low [PPh3], the reaction rate was nearly zero order in PPh3, but reactions at high [PPh3] revealed an inverse dependence of reaction rate on PPh3. The rate constants were similar in toluene, THF, and chlorobenzene. The y-intercept of a1/kobs vs [PPh3] plot displayed a primary isotope effect, indicating that the y-intercept did not simply correspond to phosphine dissociation. These data and a dependence of alkoxide racemization on [PPh3] showed that the elementary â-hydrogen elimination step was reversible. A mechanism involving reversible â-hydrogen elimination followed by associative displacement of the coordinated ketone or aldehyde by PPh3 was consistent with all of our data. This mechanism stands in contrast with the pathways proposed recently for alkoxide â-hydrogen elimination involving direct elimination, protic catalysts, or binuclear mechanisms and shows that alkoxide elimination can follow pathways similar to those for â-hydrogen elimination from alkyl complexes. Introduction The microscopic reverse of this reaction is olefin insertion into a metal hydride, which occurs in olefin hydrogenation.13 1,2 â-Hydrogen elimination and migratory insertion are com- â-Hydrogen elimination from a metal alkyl must be suppressed mon elementary reactions in both homogeneous and heteroge- to observe cross-coupling14 of main group alkyl reagents with neous catalysis. Extensive mechanistic studies have been vinyl and aryl electrophiles. conducted on â-hydrogen elimination from transition metal alkyl â-Hydrogen elimination from alkoxo and amido complexes 1 complexes. These mechanistic studies have revealed a common and the microscopic reverse of this reaction are similarly reaction pathway that requires an open coordination site and a involved in catalytic processes. These processes include transfer R transition state involving a syn coplanar arrangement of the - hydrogenations using alcohol reducing agents15-17 and cyclo- and â-carbons, the â-hydrogen, and the metal center. This isomerizations involving aldehydes.18 The microsopic reverse transition state generates an olefin hydride complex, which often of this reaction is involved in aldehyde, ketone, and imine generates the final product after olefin dissociation. hydrogenation.16,19,20 â-Hydrogen elimination from alkoxo â-Hydrogen elimination from a metal alkyl and the micro- complexes must be avoided if aromatic etherification is to scopic reverse of this reaction occurs in a variety of catalytic encompass alcohol substrates that bear a methylene or methine processes including dehydrogenation of alkanes,3-6 Mizoroki- Heck olefination,7 olefin isomerization,8 cycloisomerizations,9 (10) Collman, J. P.; Hegedus, L. S.; Norton, J. R.; Finke, R. G. In 10-12 Principles and Applications of Organotransition Metal Chemistry, 2nd ed.; and hydroformylation of internal olefins to n-aldehydes. University Science Books: Mill Valley, 1987; p 622. (11) van der Veen, L. A.; Kamer, P. C. J.; van Leeuwen, P. Angew. (1) Cross, R. J. In The Chemistry of the Metal-Carbon Bond; Hartley, Chem. Int. Ed. Engl. 1999, 38, 336-338. F. R., Patai, S., Eds.; John Wiley: New York, 1985; Vol. 2, pp 559-624. (12) Breit, B.; Winde, R.; Harms, K. J. Chem. Soc. 1997, 2681-2682. (2) Collman, J. P.; Hegedus, L. S.; Norton, J. R.; Finke, R. G. In (13) Collman, J. P.; Hegedus, L. S.; Norton, J. R.; Finke, R. G. In Principles and Applications of Organotransition Metal Chemistry, 2nd ed.; Principles and Applications of Organotransition Metal Chemistry, 2nd ed.; University Science Books: Mill Valley, 1987; pp 383-388. University Science Books: Mill Valley, 1987; p 523. (3) Crabtree, R. H.; Mellea, M. F.; Mihelcic, J. M.; Quirk, J. M. J. Am. (14) Hegedus, L. S. Transition Metals in the Synthesis of Complex Chem. Soc. 1982, 104, 107-113. Organic Molecules; University Science Books: Mill Valey, CA, 1994. (4) Felkin, H.; Fillebeen-Khan, T.; Gault, Y.; Holmes-Smith, R.; (15) Uematsu, N.; Fujii, A.; Hashiguchi, S.; Ikariya, T.; Noyori, R. J. Zakrzewski, J. Tetrahedron Lett. 1984, 25, 1279-1282. Am. Chem. Soc. 1996, 118, 4916-4917. (5) Liu, F.; Pak, E. B.; Singh, B.; Jensen, C. M.; Goldman, A. S. J. Am. (16) Noyori, R.; Hashiguchi, S. Acc. Chem. Res. 1997, 30,97-102. Chem. Soc. 1999, 121, 4086-4087. (17) Mestroni, G.; Zassinovich, G.; Camus, A.; Martinelli, F. J. Organo- (6) Liu, F. C.; Goldman, A. S. Chem. Commun. 1999, 655-656. met. Chem. 1980, 198,87-96. (7) Crisp, G. T. Chem. Soc. ReV. 1998, 27, 427-436. (18) Montgomery, J. Acc. Chem. Res. 2000, 33, 467-473. (8) For a recent example see: Wakamatsu, H.; Nishida, M.; Adachi, N.; (19) Palmer, M. J.; Wills, M. Tetrahedron: Asymmetry 1999, 2045- Mori, M. J. Org. Chem. 2000, 65, 3966-70 and references therein. 2061. (9) Trost, B. M.; Krische, M. J. Synlett 1998,1-16. (20) James, B. R. Chem. Ind. 1995, 62, 167-180. 10.1021/ja010417k CCC: $20.00 © 2001 American Chemical Society Published on Web 07/06/2001 â-H Elimination from Square Planar Iridium Alkoxide Complexes J. Am. Chem. Soc., Vol. 123, No. 30, 2001 7221 hydrogen R to oxygen.21,22 Despite being common steps of Scheme 1 catalytic processes, direct observations of â-hydrogen elimina- tion from alkoxo and amido complexes are much less common than those from alkyl complexes,23-29 and â-hydrogen elimina- tion from alkoxo complexes is not well defined mechanistically. Evidence for â-hydrogen elimination from alkoxides is indirect in most cases. Yet, a few mechanistic studies have been conducted on isolated alkoxo complexes that cleanly generate aldehyde or ketone and metal hydride product, and in each case the mechanism has been proposed or shown to be distinct from the conventional mechanism for â-hydrogen elimination from alkyls. In one case, alkoxide â-hydrogen elimination from a 16-electron square planar complex [(DPPE)Pt(OMe)2] oc- curred.28 This reaction was faster than â-hydrogen elimination drogen elimination that are similar to those of the 18-electron from classic platinum dialkyls with the same ligand. It was Ir(III) complexes or to those of classic square planar alkyl proposed that an 18-electron aldehyde hydride complex is complexes, we studied alkoxo complexes based on the Vaska formed as an intermediate. However, chelation of the phosphine fragment. Atwood had reported previously some of the alkoxo prevented detailed data on possible ligand dissociation paths. complexes we used for the study. He had shown that they were 31 Partial dissociation of the chelating phosphine is generally isolable and would generate iridium hydride upon thermolysis. undetectable by kinetic studies. Moreover, we had recently provided some mechanistic data on 24 More detailed mechanistic data have been obtained on â-hydrogen elimination from related amido complexes, al- â-hydrogen elimination from saturated Ir(III) alkoxo complexes, lowing comparisons between the mechanism for â-hydrogen and these studies revealed unusual mechanisms.23,26,27 In two elimination from alkoxo and amido complexes. cases the reaction occurred in the presence of added alcohol,23,27 We report our results from this study, which provided an and the order of the reaction in added alcohol was positive and unusually detailed reaction path for the â-hydrogen elimination. noninteger. Thus, the authors could not pinpoint a transition This pathway involves a reversible, elementary â-hydrogen state that contains the number of methanol groups in the elimination step. An unexpected influence of phosphine con- experimental rate equation, but proposed a structure with centration on reaction rate and racemization of the alkoxide hydrogen bonding involving a variable number of alcohols. The complex uncovered the reversibility of this step. The isotope other example of â-hydrogen elimination from an Ir(III) alkoxo effect on the y-intercept of a plot correlating 1/kobs with complex involved a dinuclear transition state formed from phosphine concentration also revealed this reversibility. catalytic amounts of an iridium triflate.26 Results One study of rhenium alkoxo complexes probed â-hydrogen elimination indirectly.30 In this case, the data supported a 1. Synthesis and Thermolysis of Vaska-Type Alkoxo mechanism that is similar to the typical one for â-hydrogen Complexes. The synthesis and thermolysis of Vaska-type elimination from alkyl complexes. [Cp*Rh(NO)(PPh3)OR] alkoxide complexes are summarized in Scheme 1. The isopro- catalyzed the racemization of alcohols, and this racemization poxide was prepared previously by Atwood,31,32 who first was proposed to occur by â-hydrogen elimination of the rhenium showed that it underwent â-hydrogen elimination. In our hands, alkoxide. The rate of racemization depended inversely on sodium alkoxides reacted with Vaska’s complex within an hour phosphine concentration. This information implied that â-hy- in THF solvent to form stable, monomeric, terminal alkoxo or drogen elimination was preceded by phosphine dissociation and benzyloxo complexes bearing â-hydrogens in 46-84% yield.
Details
-
File Typepdf
-
Upload Time-
-
Content LanguagesEnglish
-
Upload UserAnonymous/Not logged-in
-
File Pages8 Page
-
File Size-