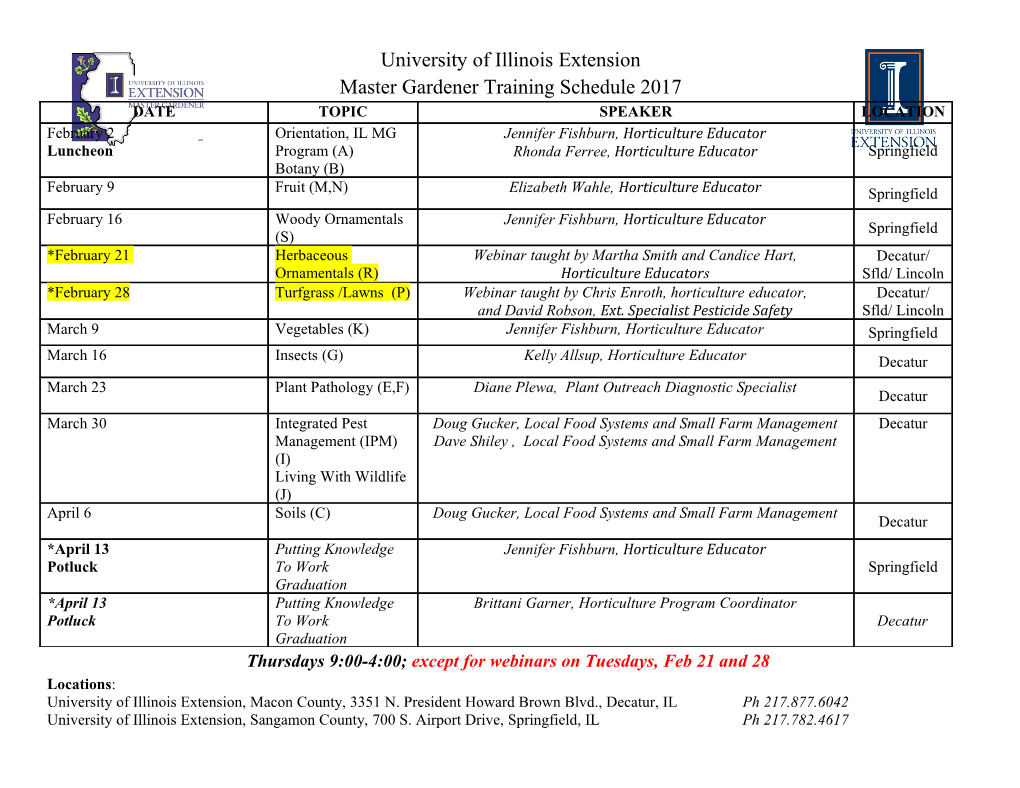
Chemical Engineering Science 156 (2016) 1–10 Contents lists available at ScienceDirect Chemical Engineering Science journal homepage: www.elsevier.com/locate/ces Optimized polybutylene terephthalate powders for selective laser beam melting Jochen Schmidt a,b,n, Marius Sachs a,b, Stephanie Fanselow a,b, Meng Zhao c, Stefan Romeis a,b, Dietmar Drummer b,c, Karl-Ernst Wirth a,b, Wolfgang Peukert a,b a Institute of Particle Technology, Friedrich-Alexander-Universität Erlangen-Nürnberg, Cauerstraße 4, D-91058, Erlangen, Germany b Interdisciplinary Center for Functional Particle Systems, Friedrich-Alexander-Universität Erlangen-Nürnberg, Haberstraße 9a, D-91058, Erlangen, Germany c Institute of Polymer Technology, Friedrich-Alexander-Universität Erlangen-Nürnberg, Am Weichselgarten 9, D-91058, Erlangen, Germany HIGHLIGHTS GRAPHICAL ABSTRACT Process chain for producing poly- butylene terephthalate (PBT) pow- ders for selective laser beam melting (LBM). Processability of powders is assessed by characterization of sintered thin layers. Influence of particle shape and size on processability in LBM is studied. Good LBM processability is observed for PBT powders of good flowability. Device quality is remarkably influ- enced by powder flowability and bulk density. article info abstract Article history: Additive manufacturing processes like selective laser beam melting of polymers (LBM) are established for Received 7 April 2016 production of prototypes and individualized parts. The transfer to serial production currently is hindered Received in revised form by the limited availability of polymer powders with good processability. 23 July 2016 Within this contribution the effect of powder properties, such as particle size, shape and flowability Accepted 5 September 2016 on the processability in LBM and their influence on device quality is exemplified for polybutylene ter- Available online 5 September 2016 ephthalate (PBT) materials. A process chain for the production of spherical polymer microparticles has Keywords: been developed to obtain PBT powder materials. The process chain consists of three steps: first, polymer Poly butylene terephthalate microparticles are produced by wet grinding. Second, the particle shape is engineered by rounding in a Wet grinding heated downer reactor to improve the flowability of the product. Third, a further improvement of Downer reactor flowability of the still cohesive spherical PBT particles is realized by dry coating with fumed silica. Dry particle coating Flowability Moreover, properties of the PBT powders obtained along the process chain are thoroughly char- Selective laser beam melting acterized with respect to structure and crystallinity by infrared spectroscopy, X-ray diffraction and dif- ferential scanning calorimetry. The effect of flowability, shape and bulk density on the powders’ pro- cessabilities in LBM is assessed by characterization of the quality of thin layers built in a LBM device. It is demonstrated that the device quality is strongly determined by particle properties: powders of good flowability and high bulk density are mandatory to obtain dense devices. & 2016 Elsevier Ltd. All rights reserved. n Corresponding author at: Institute of Particle Technology, Friedrich-Alexander-Universität Erlangen-Nürnberg, Cauerstraße 4, D-91058, Erlangen, Germany. E-mail addresses: [email protected] (J. Schmidt), [email protected] (M. Sachs), [email protected] (S. Fanselow), [email protected] (M. Zhao), [email protected] (S. Romeis), [email protected] (D. Drummer), [email protected] (K.-E. Wirth), [email protected] (W. Peukert). http://dx.doi.org/10.1016/j.ces.2016.09.009 0009-2509/& 2016 Elsevier Ltd. All rights reserved. 2 J. Schmidt et al. / Chemical Engineering Science 156 (2016) 1–10 Nomenclature TMeasured measured temperature (downer reactor) / °C Tmelt melting temperature / °C ° af final particle radius (viscous flow sintering model, TSet set temperature (heating of downer reactor) / C Kirchhof et al. (2009)) / m vs scan speed / m/s df focal diameter / m vtip stirrer tip speed / m/s 2 dGM Ebeam laser energy input / J/m grinding media dia- x particle size / m meter / m LBM laser beam melting Greek letters PL laser power / W PA polyamide ΔHf (mass-specific) heat of fusion / J/kg PBT polybutylene terephthalate η viscosity / Pa s PEEK poly ether ether ketone λ wavelength / m – 3 Q3 volume sum distribution / ρGM grinding media density / kg/m RRMS root mean square roughness s surface tension / N/m SE stress energy / J θ angle of diffraction / ° t sintering time / s 1. Introduction further modification. Therefore, a process route for production of spherical polymer microparticles with good flowability consisting Additive manufacturing methods allow the tool-free and of wet grinding (Schmidt et al., 2012; Wolff et al., 2014; Schäfer formless production of complex structures. Especially selective et al., 2016), rounding of the irregular-shaped comminution pro- laser beam melting (LBM) of polymers as a generative powder- duct in a heated downer reactor and a dry coating step (Pfeffer and beam-based manufacturing process is promising. Several et al., 2001; Yang et al., 2005; Blümel et al., 2015) has been pro- years ago, generative manufacturing methods have been almost posed and successfully applied to polystyrene (Schmidt et al., exclusively applied in the production of parts at low piece num- 2014). Compared to dry grinding, wet grinding of polymers allows bers in applications where mechanical properties of the built de- for much smaller product particles giving the opportunity to lower vices typically were of minor importance (rapid prototyping). With the size range of LBM materials. If appropriate process conditions, the advent of additive manufacturing, i.e. the application of gen- i.e. low-viscous solvents and a moderately reduced process tem- erative methods in mass production – commonly referred to as ‘3D perature are chosen, particles smaller than 10 mm(x50,3) are printing’- new requirements with respect to process stability and readily obtained for a variety of engineering and high performance robustness, mechanical properties of the devices, as well as new plastics. For brittle, amorphous polymers like polystyrene even demands concerning the powder materials used in beam- and smaller product particles (x50,3o2 mm) can be produced (Schmidt powder-based additive manufacturing methods have been defined et al., 2012). LBM materials of small particle size are desirable (Kruth et al., 2003; Wendel et al., 2008; Drummer et al., 2010; because they allow for higher surface qualities (lower surface Goodridge et al., 2012; Schmidt et al., 2014; Wohlers, 2014; roughness) of the obtained parts and better resolution (Kruth Schmid, 2015; Witt, 2016). Preferentially semi-crystalline ther- et al., 2003). However, the commercially available polymer pow- moplasts are applied in the manufacture of functional components ders for additive manufacturing are so far in the size range of 50– via LBM because of their improved mechanical properties as 70 mm(x50,3) due to concerns regarding the processability: with compared to amorphous polymers (Wohlers, 2014; Schmid, 2015). smaller particles inhomogeneous coating during powder deposi- Currently, the spectrum of commercially available LBM powder tion in LBM due to poor flowability may occur. We demonstrate materials is very limited, about 95% of the total market share is that these problems can be overcome by rounding the irregular made up of polyamide (PA) – based materials. PA powders are shaped comminution product and dry coating (Pfeffer et al., 2001) typically produced by bottom-up approaches (c.f. emulsion poly- of the obtained spherical particles. By dry coating the surface merization) or precipitation processes are applied (Wohlers, 2014; roughness is increased on the nanoscale leading to an overall Schmid, 2015). Besides PA, polystyrene and polypropylene as well decrease of interparticulate van der Waals forces (Rumpf, 1974; as thermoplastic elastomers or PEEK (poly ether ether ketone) are Zhou et al., 2003; Götzinger and Peukert, 2004; Linsenbühler and available (Goodridge et al., 2012; Wohlers, 2014). In addition to the Wirth, 2005; Zhou and Peukert, 2008). aforementioned bottom-up processes, also top-down approaches Within this contribution the process chain is applied to pro- like cryogenic (dry) grinding (Wilczek et al., 2004; Bertling and duce spherical polybutylene terephthalate (PBT) powders of good Eloo, 2009), wet grinding of polymers (Schmidt et al., 2012), co- flowability and processability. To our knowledge at present opti- extrusion (Schmid, 2015) or melt emulsification (Fanselow et al., mized PBT powders are not available for laser beam melting of 2016) have been proposed to produce polymer microparticles. polymers. However, this semi-crystalline thermoplastic shows To further extend the applications of LBM new and cheap good mechanical properties (e.g. impact resistance) and, thus, is of materials with good processability must be supplied: device potential interest for LBM in a variety of applications, e.g. in the quality, LBM processability and powder properties (Blümel et al., automotive industries. The PBT product particles are thoroughly 2015; Ziegelmeier et al., 2015) are directly connected. Moreover, characterized with respect to both, powder properties and poly- innovative and versatile processes being applicable to a wide mer structure: Powder flowability is assessed by means of tensile variety of thermoplasts
Details
-
File Typepdf
-
Upload Time-
-
Content LanguagesEnglish
-
Upload UserAnonymous/Not logged-in
-
File Pages3 Page
-
File Size-